Background of the invention
Field of the invention
[0001] The invention relates to well testing in general and in particular to a method for
downhole measurements and recording of data from a multiple layered formation of an
oil and gas well and for estimating individual permeabilities and skin factors of
the layers using the recorded data.
Description of the prior art
[0002] The estimation of parameters of stratified layers without crossflow of an oil and
gas well is not a new problem. Over the years, many authors have investigated the
behavior of layered reservoirs without cross flow. Much work has been done on estimating
parameters of layered reservoirs because they naturally result during the process
of sedimentation. Layered reservoirs are composed of two or more layers with different
formation and fluid characteristics.
[0003] One of the major problems for layered reservoirs is the definition of the layers.
It has been found that it is essential to integrate all logs and pressure transient
and flowmeter data in order to determine flow capacities, skin factors, and the pressure
of individual layers. This invention relates primarily to two-layer reservoirs with
a flow barrier between the layers (without crossflow). The production is commingled
at the wellbore only.
[0004] During a buildup test, fluid may flow from the high pressure zone to the low pressure
zone through the wellbore as a result of a differential depletion. The crossflow problem
becomes more severe if the drainage radius of each zone is different. Wellbore crossflow
could occur while the pressure is building up. A straight line may be observed on
the Horner plot. This behavior has been observed many times in North Sea reservoirs
Lefkovits, H.C. et al.: "A Study of the Behavior of Bounded Reservoirs Composed of
Stratified Layers" Soc. Pet. Eng. J. (March 1961) 43-58; Trans., AIME, 222.
[0005] The crossflow problem has been overlooked by the prior art because in many instances
the pressure data itself does not reveal any information about the wellbore crossflow.
Furthermore, the end of the wellbore crossflow between the layers cannot be determined
either quantitatively or qualitatively. If fluid segregation in the tubing and wellbore
geometry is added to the complication mentioned above, the buildup tests from even
two-layer reservoirs without crossflow cannot easily be analyzed.
[0006] This invention relates to the behavior of a well in an infinite two-layered reservoir.
If the well has a well-defined drainage boundary (symmetrics about the well axis for
both layers), and if a well test is run long enough, the prior art has shown that
it is possible to estimate the individual layer permeabilities and an average skin.
However, cost or operational restrictions can make it impractical to carry out a test
of sufficient duration to attain a pseudo steady-state period. Moreover, even if the
test is run long enough, an analyzable pseudo steady-state period may not result because
of non-symmetric or irregular drainage boundaries for each layer. It is also difficult
to maintain a constant production rate long enough to reach a pseudo steady-state
period.
[0007] A major problem for layered systems not addressed by the prior art is how to estimate
layer permeabilities, skins, and pressures from conventioal well testing. In practice,
the conventional tests (drawdown and/or buildup) only reveal the behavior of a two-layer
formation which cannot be distinguished from the behavior of a single-layer formation
even though a two-layer reservoir has a distinct behavior without wellbore storage
effect. There are, of course, a few special cases for which the conventional tests
will work.
[0008] The effect of wellbore storage on the behavior of the layered reservoirs is more
complex than that of single-layer reservoirs. First, the wellbore storage may vary
according to the differences in flow contribution of each layer. Second, it has been
observed that it takes longer to reach the semilog straight line than that of the
equivalent single-layer systems.
[0009] It is important for the operator of an oil and gas well having a multiple layer reservoir
to be able to determine the skin factor, s, and the permeability, k, of each layer
of the formation. Such information aids the operator in his determination of which
zone may need reperforation or acidizing. Such information may also aid the operator
to determine whether loss of well production is caused by damage to one layer or more
layers (high skin factor) as distinguished from other reasons such as gas saturation
buildup. Reperforation or acidizing may cure damage to the well while it will be useless
for a gas saturation buildup problem.
Identification of objects of the invention
[0010] It is a general object of the invention to provide a well test method to estimate
multi-layered reservoir parameters.
[0011] It is a more specific object of the invention to provide a well test method to estimate
uniquely the permeability k and the skin factor s for each layer in a multiple layer
reservoir.
Summary of the invention
[0012] According to the invention, a well test method for uniquly estimating permeability
and skin factor for each of at least two layers of a reservoir includes the positioning
of a logging tool of a logging system at the top of the upper layer of a wellbore
which traverses the two layers. The logging system has means for measuring downhole
fluid flow rate and pressure as a function of time. The surface flow rate of the well
is changed from an initial flow rate at an initial time, t
1, during a first time interval. The downhole fluid flow rate, q
i(t), and downhole pressure, p
l(t), are measured and recorded during the first interval, t, to
2, at the top of the upper layer.
[0013] The logging tool is then positioned to the top of the lower layer where the downhole
flow rate, q
12, from the top of the lower layer is measured and recorded if possible at a stabilized
flow. The surface flow rate is then changed at time t
3, to another flow rate. The downhole fluid flow rate, q
22(t), and downhole pressure, p
2(t), are measured and recorded during the second interval, t
3 to t
4, at the top of the lower layer.
[0014] The functions k and 5 are determined, where

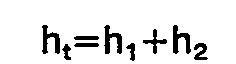

where
k1=permeability of upper layer
k2=permeability of lower layer
h,=known thickness of upper layer
h2=known thickness of lower layer

by matching the measured change in downhole pressure, p1(t), with the convolution of the measured fluid flow rate ql(t) and an influence function Δpsf(t) which is a function of combined-layerred permeability, k, and skin effect s.
[0015] The permeability k
2, of the lower layer and the skin factor, s
2, of the lower layer are determined by matching the measured fluid flow rate, q
22(t), with the convolution of the measured changed in downhole pressure, Δp
2(t)=p
1(t
3)-p
2(t), and an influence function, f(t), which is a function of the lower layer permeability,
k
2, and skin factor, s
2. The parameters k
1 and s
1 for the first layer are determined from estimates of k
2, s
2 and k and s.
[0016] Testing regimes are defined for non-flowing wells and for flowing wells. Estimation
methods are presented for matching measured values of pressure and flow rate with
calculated values, where the calculated value changes as a result of changes in the
parameters to be estimated, k and s.
Brief description of the drawings
[0017] The objects, advantages and features of the invention will become more apparent by
reference to the drawings which are appended hereto and wherein like numerals indicate
like parts and wherein an illustrative embodiment of the invention is shown of which:
Figure 1 illustrates schematically a two layer reservoir in which a logging tool of
a wireline logging system is disposed at the top of the upper producing zone;
Figure 2 illustrates the same system and formation as that of Figure 1 but in which
the logging tool of the wireline logging system is disposed at the top of the lower
producing zone;
Figure 3A illustrates the sequential flow rate profile for a new well according to
the invention;
Figure 3B illustrates the downhole pressure profile which results from the flow rate
profile of Figure 3A.
Figure 4A illustrates the sequential flow rate profile for a producing well according
to the invention;
Figure 4B illustrates the downhole pressure profile which results the flow rate profile
of Figure 4A;
Figure 5 is a graph of measured downhole pressure as a function of time of a synthetic
drawdown test according to the invention; and
Figure 6 is a graph of measured downhole flow rates as a function of time of a synthetic
drawdown test corresponding to the measued pressure of Figure 5.
Description of the invention
[0018] Figures 1 and 2 illustrates a two layered reservoir, the parameters of permeability,
k, and skin factor s, of each layer of which are to be determined according to the
method of this invention. Although a two-layered reservoir is illustrated and considered,
the invention may be used equally advantageously for reservoirs of three or more layers.
A description of a mathematical model of the reservoir is presented which is used
in the method according to the invention.
Mathematical model
[0019] The reservoir model of Figures 1 and 2 consists of two layers that communicate only
through the wellbore. Each layer is considered to be infinite in extent with the same
initial pressure.
[0020] From a practical point of view, it is easy to justify an infinite-acting reservoir
if only the data is analyzed that is not affected by the outer boundaries. However,
often a differential depletion will develop in layered reservoirs as they are produced.
It is possible that each layer may not have the same average pressure before the test.
It is also possible that each layer may have different initial pressures when the
field is discovered. The method used in this invention is for layers having equal
initial pressures, but the method according to the invention may be extended for the
unequal initial pressure case.
[0021] It is assumed that each layer is homogeneous, isotropic, and horizontal, and that
it contains a slightly compressible fluid with a constant compressibility and viscosity.
[0022] The Laplace transform of the pressure drop for a well producing at a constant rate
in a two-layered infinite reservoir is given by

where:
η=hydrauIic diffusivity
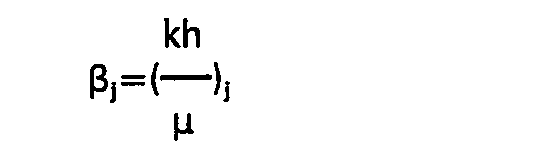
z=Laplace image space variable
n1=number of layers=2
[0023] The other symbols are defined in Appendix A at the end of this description where
the nomenclature of symbols is defined.
[0024] The Laplace transform of the production rate for each layer can be written as:

[0025] Eqs. 1 and 2 give the unsteady-state pressure distribution and individual production
rate, respectively, for a well producing at a constant rate in an infinite two-layered
reservoir.
[0026] For drawdown or buildup tests, Eq. 1 cannot be used directly in the analysis of wellbore
pressure because of the wellbore storage (afterflow) effect (unless the semilog straight
line exists). However, most studies on layered reservoirs have essentially investigated
the behavior of Eq. 1 for different layer parameters. The principal conclusions of
these studies can be outlined as follows:
1. From buildup or drawdown tests, an average flow capacity and skin factor can be
estimated for the entire formation.
2. The individual flow capacities can be obtained if the stabilized flow rate from
one of the layers is known and if the skin factors are zero or equal to one another.
[0027] Estimating layer parameters by means of an optimum test design has been investigated
by the prior art using a numerical model similar to that of equation 1 except that
skin factors were not included. Such prior art shows that there are serious problems
with observability and the question of wellposedness of the parameter estimation for
layered reservoirs. Dogru, A. H. and Steinfeld, J. H., "Design of Well Tests to Determine
the Properties of Stratified Reservoirs", Proceedings of the 5th SPE Symposium on
Reservoir Simulation, Denver, CO, February 1-2, 1979.
[0028] The basic problem of prior art methods for estimating layer parameters is that the
pressure data are not sufficient to estimate the properties of layered reservoirs.
The invention described here is for a two-step drawdown test with the simultaneously
measured wellbore pressure and flow rate data which provides a better estimate for
layer parameters than prior art drawdown or buildup tests. Eqs. 1 and 2 will be used
to describe the behavior of two-layered reservoirs.
Wellbore pressure behavior
[0029] Certain aspects of the pressure solution for two-layer systems were discussed in
the previous section; basically the constant rate solution was presented. In reality,
the highly compressible fluid in the production string will affect this solution.
This effect is usually called wellbore storage or afterflow, depending on the test
type. It has been a common practice to assume that the fluid compressibility in the
production string remains constant during the test. Strictly speaking, this assumption
may only be valid for water or water-injection wells. The combined effects of opening
or closing the wellhead valve and two-phase flow un the tubing will cause the wellbore
storage to vary as a function of time. In many cases, it is difficult to recognize
changing wellbore storage because it is a gradual and continuous change. Nevertheless,
the constant wellbore storage case is considered here as well.
[0030] The convolution integral (Duhamel's theorem) is used to derive solutions from Eq.
1 for time-dependent wellbore (inner boundary) conditions. For example, the constant
wellbore storage case is a special time-dependent boundary condition. For a reservoir
with an initially constant and uniform pressure distribution, the wellbore pressure
drop is given by


where
Δpwf=Pf-pwf for drawdown tests
Δpsr=p1-Psf for drawdown tests
Δpwf=pws-pwf for buildup tests
ΔPsf=Psf-pwf for buildup tests
Δps=pressure drop caused by skin
psf=sandface pressure of a well producing at constant rate
qD<tD)=qsf(t)/qt
q.f=sandface flow rate
qt=reference flow rate
'=indicates derivative with respect to time
[0031] The Laplace transform of Δp
wf is given by

[0032] If the wellbore storage is constant, q
o can be expressed as

[0033] Substitution of the Laplace transform of Eq. 5 and Eq. 1 in Eq. 4 yields the wellbore
pressure solution for the constant wellbore storage case.
[0034] Wellbore storage effects for layered reservoirs may be expressed as:

where a is dependent on reservoir and wellbore fluid properties.
[0035] This condition can be interpreted as a special variable wellbore storage case. It
is also possible that for some wells, Eq. 6 describes wellbore storage phenomena far
better than Eq. 5. If the sandface rate is measured with available flowmeters, it
is not necessary to guess the wellbore storage behavior of a well.
[0036] A drawdown test with a periodically varying rate with a different period is considered
in order to increase the sensibility of pressure behavior to each layer parameter.
This case is expressed as:

where T=period.
Identifiability of layer parameters in well test analysis
[0037] The problem of identifiability has received considerable attention in history matching
by the prior art. The purpose here is to give an identifiability criterion to nonlinear
estimation of layer parameters, Dogru, A. H., Dixon, T. N., and Edgar, T. F: "Confidence
Limits on the Parameters and Prediction of Slightly Compressible, Single-Phase Reservoirs",
Soc. Pet. Eng. J. (Feb. 1977) 42-56. The identifiability principles given here are
very general, and are also applied to other similar reservoir parameter estimations.
[0038] The main objective of this section is to estimate layer parameters using the model
presented by Eq. 1 and measured wellbore pressure data. For convenience, it is assumed
that the measured pressure is free of errors.
[0039] Suppose that wellbore pressure, p°, is measured m times as a function of time from
a two-layered reservoir. It is desired to determine individual layer permeabilities
and skin factors from the measured data by minimizing:

where
pj=measured pressure
η=calculated pressure as a function of time, and β
β=(k1,k2,s1,s2)τ =parameter vector
k1,k2=permeabilities of first and second layers, respectively
s1,s2=skin factors of first and second layers, respectively
m=number of measurements
[0040] Eq. 8 can also be written as:

where
r=m dimensional residual vector
Assume that β* is the true solution to Eq. 8. The necessary condition for a unique
minimum is:
1. g (β*)=0 and
2. H(β*) must be positive definite
where g the gradient vector with respect to β and H is the Hessian matrix of Eq. 8.
A positive definite Hessian, also known as the second order condition, ensures that
the minimum is unique. Furthermore, without measurement errors, or when the residual
is very small, the Hessian can be expressed as:

where A is the sensitivity coefficient matrix with mxn elements


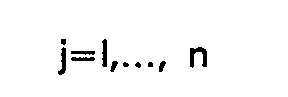
[0041] The positive definiteness of the Hessian matrix requires that all of the eigenvalues
corresponding to the system

be positive and greater than zero. If an eigenvalue of the Hessian matrix is zero,
the functional defined by Eq. 8 does not change along the corresponding eigenvector,
and the solution vector β* is not unique. Therefore, the number of observable parameters
from m measurements can be determined theoretically by examining the rank of the Hessian
matrix H, which is equal to the number of nonzero eigenvalues.
[0042] In the above analysis, it is assumed that the observations are free of any measurement
errors. In presence of such errors and limitations related with the pressure gauge
resolution, a non-zero cutoff value must be used in estimating the rank of the.Hessian.
Also, in order to compare parameters with different units, a normalization of the
sensitivity coefficient matrix can be carried out by multiplying every column of the
sensitivity coefficient matrix by the corresponding nonzero parameter value. That
is,
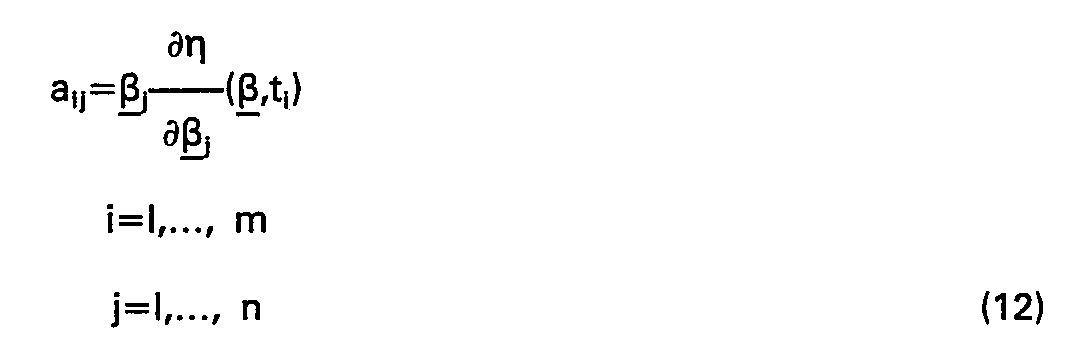
Furthermore, the largest sensitivity of the functional two parameters is along the
eigenvector corresponding to the largest eigenvalue. Each element of the eigenvector
v
j corresponds to a parameter in the n dimensional parameter space. The magnitude indicates
the relative strength of that parameter along the eigenvector v
j.
Nonlinear estimation of layer parameters from conventional transient tests
[0043] In this section an attempt is made to estimate layer parameters by minimizing Eq.
8. The eigenvalue analysis of the sensitivity coefficient is done for four cases.
1. Constant flow rate with no wellbore storage C=0,0 I/kPa (C=0,0 bbl/psi),
2. Constant flow rate with wellbore storage C=23 1/kPa (C=0.01 bbl/psi)
3. Periodically varying flow rate with a period of 0.1 hours,
4. Periodically varying flow rate with a period of 1 hour.
[0044] The pressure data for each case are generated by using Eq. 1 and Eq. 3 with the corresponding
q
D solution. Reservoir and fluid data are given in Table 1 for all these cases.
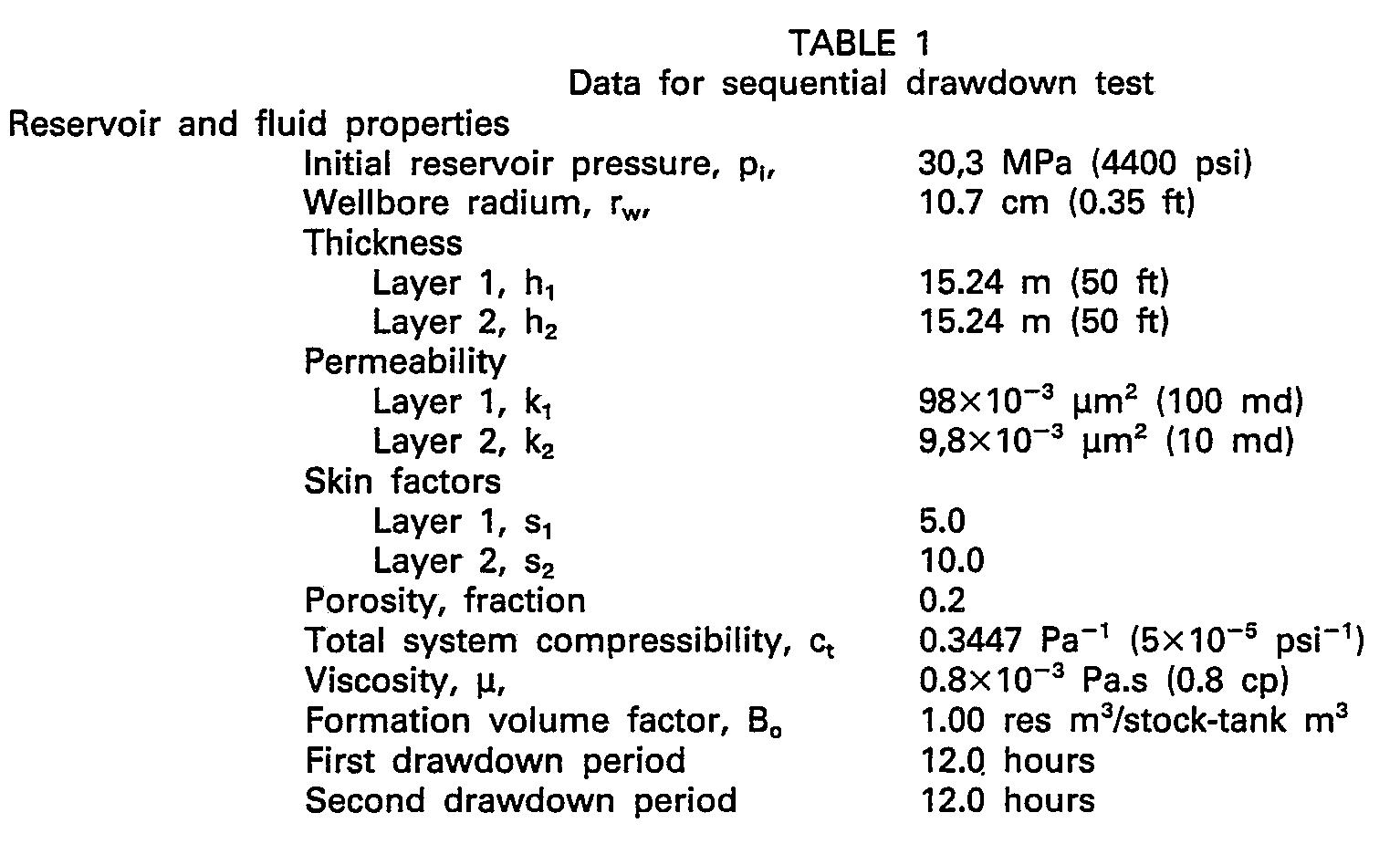
[0045] The nonlinear least squares Marquardt method with simple constraints is used for
the minimization of Eq. 8 with respect to k
1, k
2, s, and s
2.
[0046] Table 2 shows the eigenvalues of the Hessian matrix for each test.
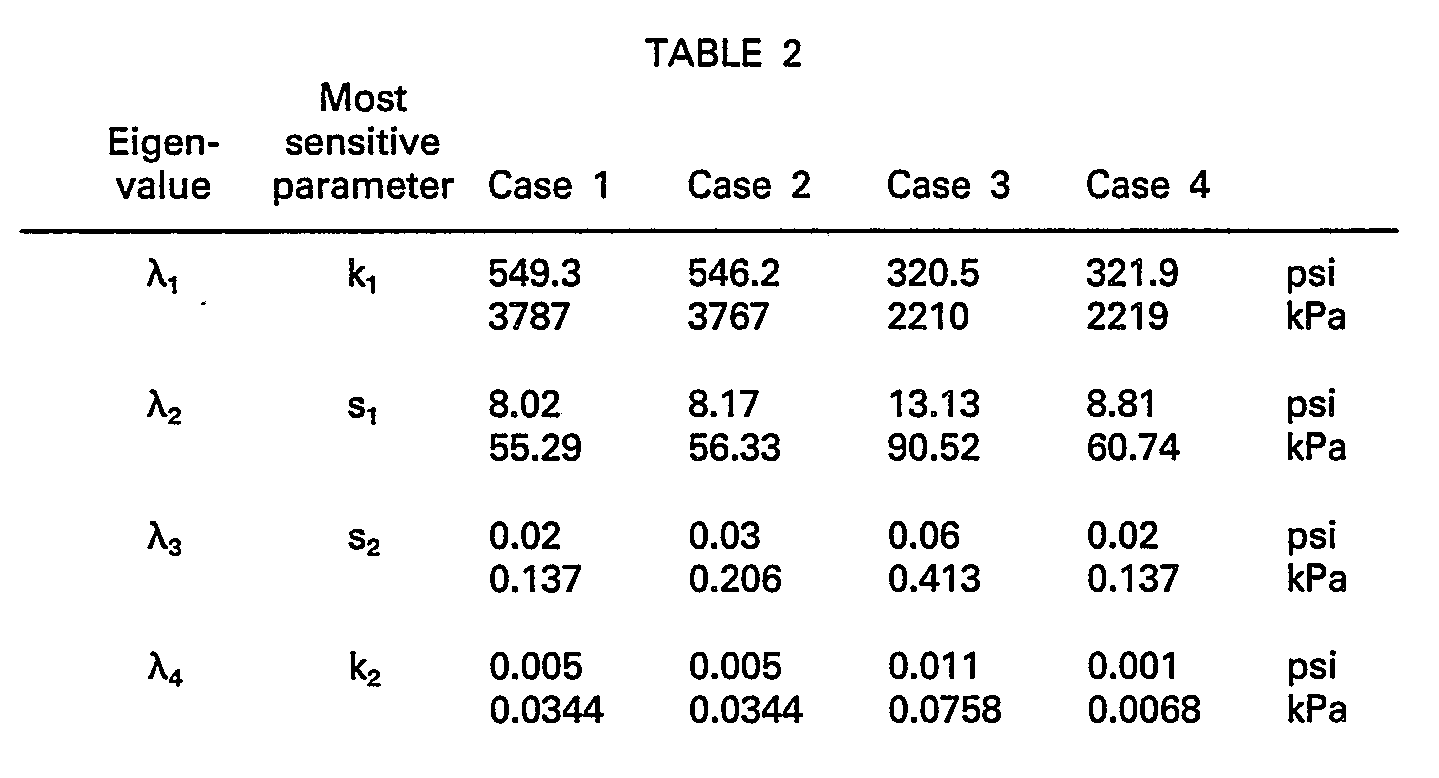
[0047] The results of Table 2 clearly indicate that in all cases only two of the eigenvalues
are greater than 6,9 kPa (1 psi). Thus, only two parameters can be uniquely estimated
from wellbore pressure data. The largest sensitive parameters are those of the high-permeability
layer.
[0048] The above analysis has also been done for different combinations of k
1, k
2, s" and s
2; and the conclusions essentially do not change. The periodical variable rate with
0.1 hours period improves the nonuniqueness problem somewhat. However, the uniqueness
problem remains the same for the estimation of k
i, k
2, s
i, and s
2 for two-layered reservoirs without crossflow.
[0049] The above analysis was also extended to a case with an unknown wellbore storage coefficient
can be estimated from wellbore pressure data if it remains constant during the test.
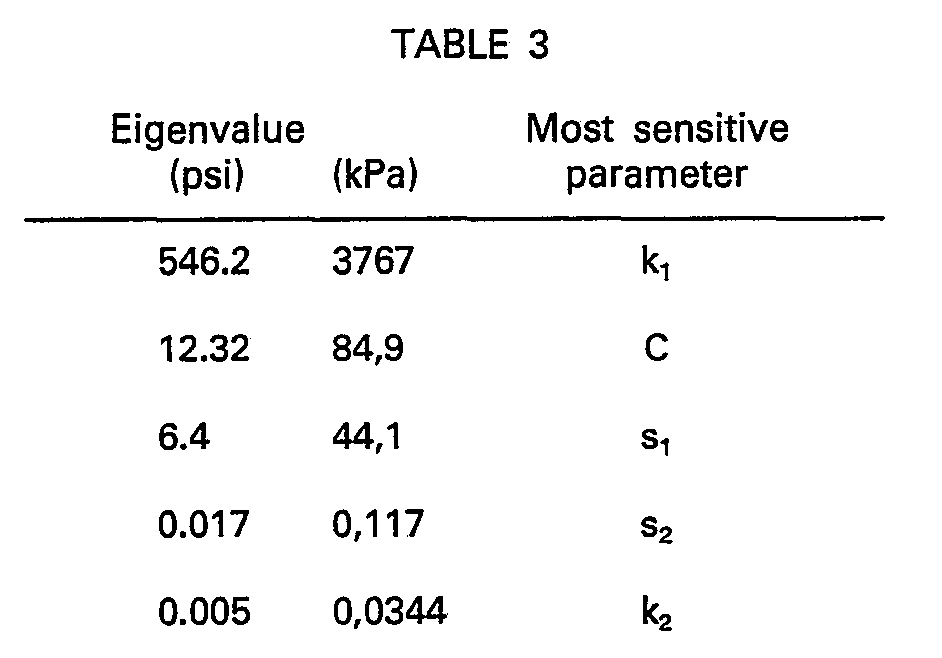
[0050] It is clear from the above discussion that using prior art methods, transient pressure
data does not give enough information to determine uniquely flow capacity and skin
factor for each individual layer.
New testing methods for layered reservoirs
[0051] As indicated above, a drawdown test is best suited for two-layered reservoirs without
crossflow. Ideally, a reservoir to be tested should be in complete pressure equilibrium
(uniform pressure distribution) before a drawdown test. In practice, the complete
pressure equilibrium condition cannot be satisfied throughout the reservoir if wells
have been producing for some time from the same formation. Nevertheless, the pressure
equilibrium condition can easily be obtained in new and exploratory reservoirs.
[0052] For developed reservoirs, it is also possible to obtain pressure equilibrium if the
well is shut in for a long time. However, in developed layered reservoirs, it is difficult
to obtain pressure equilibrium within the drainage area of a well. On the other hand,
it is very common to observe pressure differential between the layers.
[0053] In addition to these fluid flow characteristics in layered reservoirs, cost and/or
operational restrictions can make it impractical to close a well for a long time.
[0054] With respect to these different initial conditions, two drawdown test procedures
for two-layer reservoirs without crossflow are described according to the invention.
Either the initial condition or the stabilized period is important in a given test
because during the analysis, the delta pressure (p-p
base) is used for the estimation of parameters. During the test, it is not crucial to
keep the rate constant, since it is measured.
New or shut-in wells
[0055] The method according to the invention will work well for the wells in a new field
of exploratory wells. Figures 1 and 2 illustrate a two zone reservoir with a wellbore
10 extending through both layers and to the earth's surface 11. A well logging tool
14 having means for measuring downhole pressure and fluid flow rate communicates via
logging cable 16 to a computerized instrumentation and recording unit 18.
[0056] As indicated in Figure 1, the parameters k
i, s, of layer 1 and k
2, s
2 of layer 2 are desired to be uniquely estimated. One layer, such as layer 2, may
have a damaged zone which would result in a high value of s
2, skin factor of layer 2, which if known by measurement by the well operator, could
aid in decisions relating to curing low flow or pressure from the well.
[0057] For shut-in wells, before starting the test, pressure should be recorded for a reasonable
time in order to obtain the rate of pressure decline or to observe a uniform pressure
condition in the reservoir. Figure 3A shows the test procedure. First the well tool
14 of Figure 1 should be just above both producing layers. At time t
i, the well should be started to produce at a constant rate at the surface, if possible.
Although production rate does not affect the analysis, a rapid rate increase can cause
problems. A few of these are:
1. Wellbore fluid momentum effect,
2. Non-Darcy flow around the wellbore, and
3. Two-phase flow at the bottom of the well.
[0058] The third problem, which is the most important one, can be avoided by monitoring
the flowing wellbore pressure and adjusting the rate accordingly. These three complicating
factors should be avoided for all the transient tests, if possible.
[0059] During the first drawdown, when an infinite acting (without storage effect) period
is reached approximately, the test is continued a few more hours depending on the
size of the drainage area.
[0060] Next, the well tool 14 is lowered to the top of the lower zone as illustrated in
Figure 2 while monitoring measured flow rate and pressure. If there is a recordable
rate from this layer, at time t
2, the production rate should be changed to another rate. The rate can be increased
or decreased according to the threshold value of the flowmeter and the bubble point
pressure of the reservoir fluid. As can be seen in Figure 3A, the flow rate is increased.
If the rate is not recordable the test is terminated. A buildup test for further interpretation
as a single-layered reservoir could be performed.
[0061] If the rate is recordable, the test should be continued from t
3 to t
4 for another few hours until another storage-free infinite acting period is reached.
The test can be terminated at time t
4. The interpretation of measured rate and pressure data is discussed below after the
test for a producing or short shut-in well is described.
Producing or short shut-in wells
[0062] If the well is already producing at a stabilized rate, a short flow profile (production
logging) test should be conducted to check if the bottom layer is producing. If there
is enough production from the bottom layer to be detected, then as in Figure 1, the
production logging tool 14 is returned to the top of the whole producing reservoir
and the test is started by decreasing the flow rate, q
1 (t
1 as in Figure 4A to another rate, q
1(t
2). The well is allowed to continue flowing until time t
2, when the well reaches the storage-free infinite acting period. At the end of this
period, the tool string should be lowered just to the top of the bottom layer as in
Figure 2. At the time t
3, the flow rate is increased back to approximately q
1 (t
1 During the test, the rates should be kept above the threshold value of the flowmeter,
and well bore pressure should be kept above the bubble point pressure of the reservoir
fluid.
[0063] If the test precedes a short shut-in, the procedure will be the same, but the interpretation
will be slightly different.
[0064] The test procedure described above is applicable for a layer system in which the
lower zone permeability in less than the upper zone. If the upper zone is less permeable,
then the testing sequence should be changed accordingly.
Analysis of sequential drawdown test
[0065] In this section, the method according to the invention is described to estimate individual
layer parameters from measured wellbore pressure and sandface rate data. The automatic
type-curve (history) matching techniques are used to estimate k
1, k
2, sy, and s
2. In other words, Eq. 8 is minimized with respect to parameters k
1, k
2,
S1 and s
2. An automatic type-curve matching method is described in Appendix B to this description
of the invention. Unlike the semilog method, the automatic type curve matching usually
fits early time data as well as the storage-free infinite acting period if it exists
to a given model.
Analysis of the first drawdown test:
[0066] Figure 5 presents the wellbore pressure data for synthetic sequential drawdown tests,
and Figure 6 presents sandface flow rate data for the same test using the reservoir
and fluid data given in Table 1. As can be seen from Figure 6, the test is started
from the initial conditions and the well continues to produce 238 m
3/day (1,500 bbl/day) for 12 hours. For the second drawdown, the rate is increased
from 238 m
3/day (1,500 bbl/day) to 476 m
3/day (3,000 bbl/day). Figure 6 shows the total and individual flow rates from each
zone. In an actual test, during the first drawdown, only total flow rate, q
l(t), will be measured. During the second drawdown, only the rate from the bottom zone
will be measured. It is also important to record the flow rate from the lower layer
for a few minutes just before the second drawdown test.
[0067] The automatic type-curve matching approach is suitable for this purpose. If it is
applicable, the semilog portion of the pressure data should also be analyzed. In general,
type-curve matching with the wellbore pressure and sandface rate is rather straightforward.
A brief mathematical description of the automatic type-curve matching procedure is
given in Appendix B. In any case, the automatic type-curve method that is used fits
the first drawdown data to a single layered, homogeneous model. The estimated values
of and 5 are where


[0068] At the end of the first drawdown test, the rate from the bottom layer, q
12, should also be measured before starting the second drawdown test.
[0069] Strictly speaking, from the first test, k
1, k
2, s
1, and s
2 can be calculated by using deconvolution methods. However, the deconvolution process
is very sensitive to measurement errors, particularly errors in flow rate measurements.
On the other hand, the convolution process, Eq. 3 is a smoothing operation, and it
is less sensitive to measurement errors. Thus, the second drawdown test described
below almost assures an accurate estimation of the layer parameters. Furthermore,
the second transient creates enough sensitivity to the parameters of the less permeable
layer.
Analysis of the second drawdown test
[0070] During this test, the wellbore pressure for the whole system and flow rate for the
bottom layer are measured. Figures 5 and 6 present wellbore pressure and rate data
respectively for the second as well as the first drawdown. These data are analyzed
using the automatic type-curve matching method described above.
[0071] To estimate k
2 and s
2 from measured wellbore pressure and sandface rate data, the following equation is
minimized:

where
β=[k2,s2]
q221(t1)=measured sandface rate data from the bottom layer
ηi(β,t1)=computed sandface rate for the bottom layer
Two different methods can be used for the minimization of Eq. 15.
First method
[0072] The computed sandface rate of the bottom layer-for a variable total rate can be expressed
as:

In Eq. 2, Δp
wf is the measured well bore pressure during the second drawdown. The Laplace transform
of f(t) function in Eq. 16 can be expressed as (from Eq. 2):

The function f(z) in Eq. 17 is only a function of the lower layer parameters, k
2 and s
2. From the convolution of f(t) and Δp
wf(t), η(β,t) can be obtained by automatic type-curve matching. Thus, using η(β,t) and
measured q
22(t), Eq. 15 is used to estimate k
2 and s
2. The estimated values are:


These estimated values of k
2 and s
2 are somewhat lower than the actual values (k
2=10 and s
2=10) which raises the question of whether or not Eq. 16 is indeed a correct solution.
The direct solution of Eq. 16 gives correct values of the sandface flow rate for the
constant wellbore storage case.
[0073] The f(z) function is the Laplace transform of the dimensionless rate, q
o, for a well producing a constant pressure in an infinite radial reservoir. The flow
rate q
D changes very slowly with time. In other words, f(t) is not very sensitive to change
in k
2 and s
2. This ill-posedness becomes worse if the sandface rate is not accurately measured
at very early times. Thus, an alternate approach for the estimation of k
2 and s
2 is used to produce a more accurate estimate.
Second method
[0074] Eq. 16 can also be expressed as:

where
q'D=qsf/qt=total normalized rate, and Δpsf is defined by Eq. 1.
[0075] In order to compute n(β,t), the total rate, q
o, must be measured. The total rate cannot be measured unless two flowmeters are used
simultaneously. This is not practical using currently available logging tools. Thus,
q
o must be determined independently. This is not difficult since during the first drawdown,
the behavior of the wellbore storage is known. The sandface flow rate can either be
approximated by Eq. 5 or 6 or any other form. It is also important to measure total
flow rate just at the end of the second drawdown test. If the wellbore storage is
constant, the problem becomes easier. The Laplace transform of η(β,t) can be written
from Eq. 18 as,
C=wellbore storage constant
[0076] Since k and 5 are known from the first test, k
2 and s
2 can be estimated by minimizing Eq. 15 with respect to measured rate, q
22(t), and calculated rate, η(β,t), from Eq. 19.
[0077] For the test data presented by Figures 5 and 6, the estimated k
2 and s
2 are:
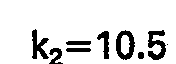
and
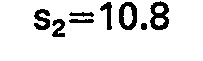
These values are very close to the actual values. The eigenvalues for k
2 and s
2 are λ
1=22,3 MPa (3244 psia) and λ2≈26 MPa (3771 psia), respectively. As can be seen from
these two eigenvalues, the sensitivity of each parameter to the model and the measurement
is very high.
[0078] Because no a priori information is assumed about the wellbore storage behavior during
the analysis of the second drawdown, the first method can be used to estimate the
lower limit of k
2 and s
2 in order to check the values calculated from the second method.
[0079] Thus there has been provided according to the invention a method for testing a well
to estimate individual permeabilities and skin factors of layered reservoirs. A novel
two-step sequential drawdown method for layered reservoirs has been provided. The
invention provides unique estimates of layer parameters from simultaneously measured
wellbore and sandface flow rate data which are sequentially acquired from both layers.
The invention provides unique estimates of the parameters distinguished from prior
art drawdown or buildup tests using only wellbore pressure data.
[0080] The invention uses in its estimation steps the nonlinear least-squares (Marquardt)
method to estimate layer parameters from simultaneously measured wellbore pressure
and sandface flow rate data. A general criterion is used for the quantitative analysis
of the uniqueness of estimated parameters. The criterion can be applied to automatic
type-curve matching techniques.
[0081] The new testing and estimation techniques according to the invention can be extended
to multilayered reservoirs. In principle, one drawdown test per layer should be done
for multilayered reservoirs. During each drawdown test, the wellbore pressure and
the sandface rate should be measured simultaneously.
[0082] The new testing technique can be generalized straightforwardly to layered reservoirs
with crossflow.
[0083] The testing method according to the invention also can be used to estimate skin factors
for each perforated interval of a well in a single layer reservoir.
[0084] If the initial pressures of each layer are different, the analysis technique has
to be slightly modified. For new wells, the initial pressure of each layer can be
obtained easily from wireline formation testers.
[0085] Nonlinear parameter estimation methods used in the testing method according to the
invention provides a means to determine the degree of uncertainty of the estimated
parameters as a function of the number of measurements as well as the number of parameters
to be estimated for a given model. Prior art graphical type-curve methods cannot provide
quantitative measures to the "matching" with respect to the quality of the measured
data and the uniqueness of the number of parameters estimated.
Appendix A
Nomenclature
[0086]
A=sensitivity matrix
AT=transpose of matrix A
a=element of matrix A
C=wellbore storage coefficient, cm3/atm
ct=system total compressibility, atm-'
Ei(-x)=exponential integral
g=gradient vector
h=layer thickness, cm
h=average thickness of a layered reservoir, cm
H=Hessian matrix
Ko=modified Bessel function of the second kind and order zero
K1=modified Bessel function of the second kind and order one
k=permeability, darcy
k=average permeability, darcy
m=number of data points
nl=number of layers in a stratified system
p=pressure, atm
pwf=flowing bottomhole pressure, atm
q=production rate, cm3/s
qsf=sandface production rate cm3/s
qt=total bottomhole flow rate, cm3/s
r=radial distance, cm
r=residual vector
rw=wellbore radius, cm
s=skin factor, dimensionless
s=average skin factor of multilayer systems
S=sum of the squares of the residuals in the least-squares method
t=time, second
v=eigenvector
z=Laplace image space variable
Greek symbols
[0087]
α=rw/√ηj,s½
β=kh/µ=transmissability, darcy - cm/cp
β=parameter vector
β*=estimate of parameter vector β
A=difference
η=k/φµc=hydraulic diffusivity, cm2/s
η=computed dependent variable
λ=eigenvalue
φ=reservoir porosity, fraction
µ=reservoir fluid viscosity, cp
T=dummy integration variable
ξ=dummy integration variable
Subscripts and superscripts
[0088]
D=dimensionless
j=layer number in a multilayer system
sf=sandface
w=wellbore
wf=flowing wellbore
-=Laplace transform of
'=derivative with respect to time
Appendix B
[0089] Type-curve matching sandface flow rate
[0090] Eq. 3 can be discretized as:

[0091] The integral in Eq. A-1 can be approximated from step t
1 to t
1+1 as

[0092] The right-hand side of Eq. A-2 can be integrated directly. Substitution of the integration
results in Eq. 3 yields

where

and the first term in Eq. A-4 is given by

In Eqs. A-3 to A-5,q
d is normalized measured sandface rate defined as

[0093] For type-curve matching the Δp
sf is model dependent. For a homogeneous single-layer system, Δp
sf is given by

[0094] The cylindrical source solution can also be used instead of the line source solution
that is given by Eq. A-7. However, the difference between the two solutions is very
small. Furthermore, for the minimization of Eq. 8, many function evaluations may be
needed. Thus, Eq. A-7 will be used. If the Laplace transform solution is used, the
minimization becomes very costly because for a given time, at least 8 function evaluations
have to be made in order to obtain △
psf(t).
[0095] In Eq. A-3, the time step is fixed by the sampling rate of the measured data. It
is preferred for the integration that the data sampling rate be less than 0.1 hours;
i.e., t
1-t
1-1<0.1 hours.
[0096] In order to estimate k and s from measured wellbore pressure and sandface flow rate
data, Eq. 8 is minimized. Eq. 8 can be written as

where
β=[k.s]T
η(β,t1)=Δpwf(t1) in Eq. (A-3)
p1(T1)=the measured wellbore pressure
As mentioned earlier, S(β) is minimized by using the Marquardt method with simple
constraints.
[0097] In the case of two-layered reservoirs, Eq. 1 should be used for Δp
sf(t) instead of Eq. A-7.