[0001] This invention relates to recording elements and recording methods.
[0002] Thin film optical recording layers using chalcogenide thin-films and amorphous to
crystalline phase transitions have been the subject of many investigations since the
early 1970's. The initial interests were focused on "erasable", and therefore reusable,
optical recording layers since the amorphous to crystalline transition is, in principle,
a reversible process. Such layers are generally prepared by a vacuum process. The
layer is amorphous when so prepared. A low power, relatively long duration laser pulse
is used to heat a local spot on the layer to below the melting point for a sufficient
length of time to cause the spots to crystallize. These crystalline spots can in turn
be heated, by a higher power, shorter duration laser, above the melting point of the
crystallized spots to randomize the structure of the spots. The layer is designed
such that upon the termination of the laser pulse the cooling rate of the heated spot
is high enough that the randomized structure is frozen to achieve an amorphous state.
[0003] Thus by adjusting the laser power and duration, the state of a selected area on the
layer can be switched between the amorphous state and the crystalline state to create
a pattern of amorphous and crystalline spots which can be used for information storage.
Since the phase transition is reversible, the pattern can be erased and replaced with
a different recorded pattern. Theoretically, this erase-write cycle can be carried
out any number of times.
[0004] A principal difficulty is that the rate of crystallization of most layers studied
is usually too low. For practical applications, it is desirable to have layers which
can be crystallized by laser pulses shorter than a microsecond (µs). Presently, few
materials have demonstrated such capabilities. For some materials with high crystallization
rates (e.g. Te-Sn alloy), the data retention times are often not adequate because
of the instability of the amorphous state.
[0005] Because of the slow crystallization of most materials, the crystallization step is
generally used as the erasure step in erasable optical recording layers. A laser spot
elongated in the direction of the laser movement is used to give an effectively long
duration laser exposure. Such long laser spots cannot be used for high density recordings.
The amorphizing step, on the other hand, is used as the recording step since this
can be achieved with short laser pulse, and hence can be done at high speed.
[0006] Very few materials are known for optical recording layers in which the above described
write-erase-write cycle is of practical use. No erasable phase-change type optical
recording layers have been commercialized.
[0007] A good deal of attention has also focused on so-called "write-once" thin film optical
recording layers. Write-once simply means that the layers can be recorded upon only
once. Such layers cannot be erased and reused for a subsequent recording.
[0008] Since thin film optical recording layers are generally amorphous when prepared, it
is desirable to use the crystallization step as the recording step in write-once layers.
However, the problem of slow crystallization prevents the achievement of high data
rates. High data rates are critical for write-once layers designed for use with computers.
[0009] European Patent Application 0184452 broadly discloses erasable optical recording
layers of various metal alloys. Information recording and erasure are said to be achieved
by switching the layers between two different crystalline states. The layers are generally
prepared in the amorphous states which have to be first converted into one of the
two crystalline states before information can be recorded. The crystallization step,
achieved by either a bulk heat-treatment or a prolonged laser exposure, is said to
have a lower reflectance than the amorphous state. The examples indicate that the
materials disclosed therein have a very low rate of crystallization. This application
further teaches that the optical recording layers disclosed therein are unsuitable
for use in the amorphous-to-crystalline transition mechanism because of the instability
of the amorphous state in general.
[0010] Another problem is that many of the chalcogen containing materials which undergo
the amorphous-to-crystalline transition mechanism are usually corrosion prone.
[0011] The problem is that the prior art has not provided write-once optical recording layers
which possess the combination of a) a crystallization rate less than 1.0 µs, b) good
corrosion resistance, c) a stable amorphous state and d) a capability of high rate,
high density recordings.
[0012] The present invention provides a recording element comprising a write-once amorphous
thin-film optical recording layer of an alloy having a composition within a polygon
in a ternary composition diagram of antimony, zinc and tin described in figure 7 herein;
wherein the polygon has the following vertices and corresponding coordinates in atom
percent:

[0013] The present invention also provides a record element having
a) a composition within the above described polygon in figure 7; and
b) a pattern of amorphous and crystalline areas.
[0014] The elements of this invention do not suffer the environmental corrosion seen in
chalcogen rich thin films. The rate of crystallization of the optical recording layers
is less than 1 µs using practical laser power. The amorphous state is very stable.
Thus, recordings on the thin film are made using the amorphous to crystalline transition
mechanism. The layers are capable of high density, high rate recordings.
[0015] Layers formed from alloy compositions outside of the defined polygon a) are crystalline
as deposited or b) crystallize too slowly to be of practical use. The layers have
an amorphous to crystalline transition temperature of at least 80°C.
[0016] Especially useful record and recording elements have alloy compositions within a
polygon in figure 7 having the following vertices and corresponding coordinates:
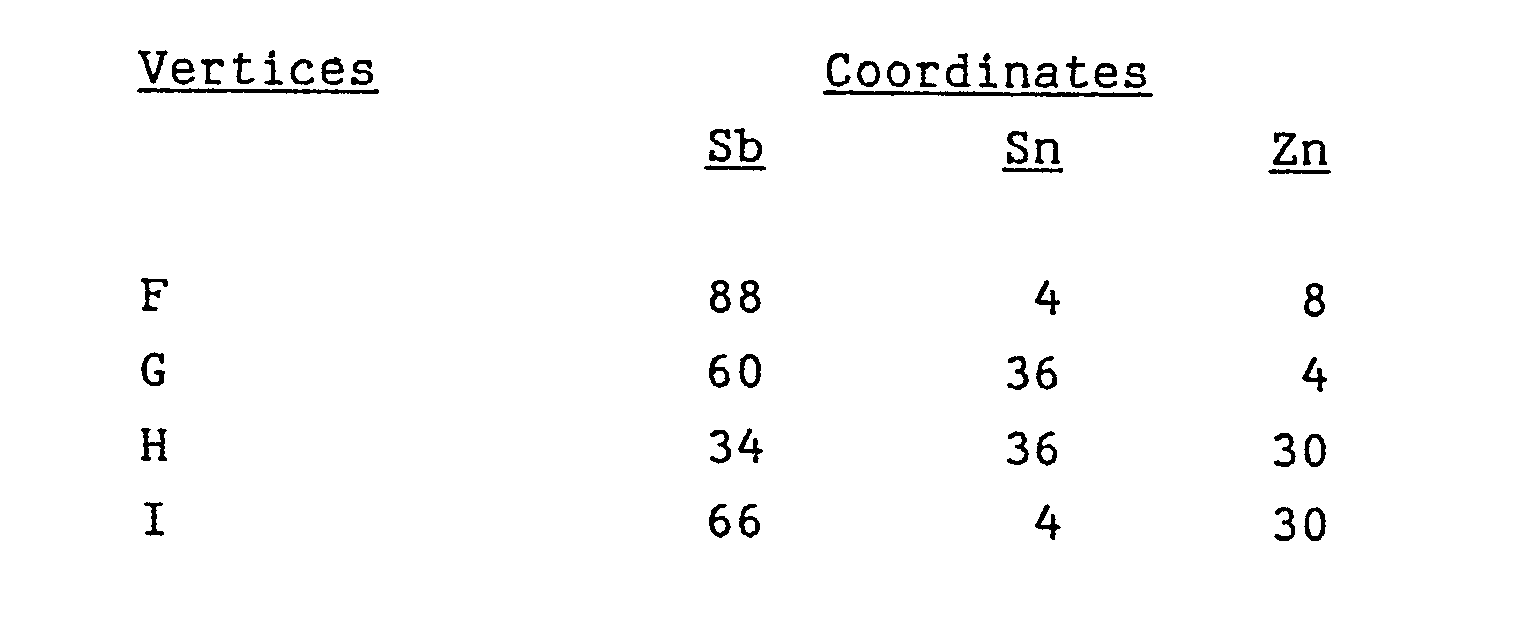
[0017] The present invention will now be described by way of example with reference to the
accompanying drawings, in which:-
Figure 1 is a description of a schematic recording and readback apparatus for using
the recording elements of the invention; and
Figure 2 is a schematic cross section of an optical recording element of this invention;
and
Figures 3, 4, 5 and 6 are curves showing some of the experimental results of the examples.
Figure 7 is a ternary composition diagram showing a polygon within which useful alloy
mixtures in the present invention are found.
[0018] Recording information on the thin film layers is achieved by focusing an information
modulated laser beam on the layer thereby forming a pattern of crystalline and amorphous
areas on the layer.
[0019] Figure 1 shows a schematic of an apparatus for recording information on an optical
recording element 16 of the invention and for playing back the recorded information
therefrom. Referring to figure 2, recording element 16 comprises an overcoat layer
41, amorphous thin film optical recording layer 42 on substrate 45. In response to
a drive signal, the intensity of a diode recording beam is modulated in accordance
with information to be recorded on thin film 42. The modulated laser beam is collected
by a lens 14 and collimated by a lens 18 and is directed by means of mirror elements
20, 23 and 24 to a lens 26 which focuses the modulated laser beam to a recording spot
28 on the film 42 as shown in Figure 1.
[0020] During recording, the element 16 is spun at a constant rate, e.g. 1800 rotations
per minute (rpm). As a result, a track of information 30 is recorded on the optical
recording layer in the form of selected crystallized areas. As recording continues,
the recording spot 28 is caused (by means not shown) to scan radially inward across
the element 16, thereby causing information to be recorded along a spiral or concentric
track that extends from an outer radius R
o to an inner radius R
i. The sizes and spacings of the recorded information marks vary in accordance with
the information content of the recording laser drive signal, as well as with radial
position on the element 16.
[0021] During the readback process, the new information bearing element 16 is spun at the
same rate as it was spun during the recording process. A laser beam 22 from a readout
laser is expanded in diameter by means of lenses 34 and 36. The optical path of the
readout laser beam is folded by a beam splitter 21 and mirrors 23 and 24 so that the
readout laser beam is focused to a playback spot on the element 16 by the high numerical
aperture lens 26. The element 16 is assumed to be of the reflective type so that the
radiation forming the playback spot is reflected back through the high numerical aperture
lens 26 after interacting with the information marks recorded on the optical element
16. A lens 36 directs reflected laser radiation which has been diverted by the prism
beamsplitter onto a detector 40 which produces an electrical playback signal in response
to temporal variations (contrast) in the irradiance of the reflected laser radiation
falling on the detector.
[0022] The amorphous thin film optical recording layers of this invention are written upon
with a coherent beam of electromagnetic radiation of sufficient energy to convert
selected portions of the amorphous film 42 to a crystalline state. In the present
invention the amorphous thin film optical recording layers are of sufficient sensitivity
that laser powers of 2 to 10 mW at laser pulsewidth of 40 to 100 nanoseconds provides
sufficient energy to make the conversion.
[0023] Recordings on the amorphous thin film were made with a static pit tester.
[0024] The static pit tester provides automated facilities in which a microcomputer controls
the sample position, the laser power and the laser pulse width. Each recording layer
is exposed with a 830 nanometer laser diode in the static pit tester to produce a
matrix of spots in which the laser power is varied from 4 to 12 mW and the pulse width
varied from 40 to 30,000 nanoseconds. The suitability of the recording layer for optical
recording is determined by measuring the change in reflection between the exposed
and unexposed areas of the sample, i.e. between the crystalline and amorphous states.
[0025] This reflection change is expressed as recording contrast, CT, by the following definition:
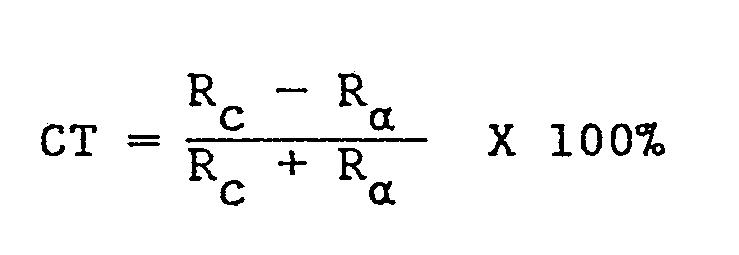
wherein R
c and R
α are the reflectances of the crystalline and the amorphous states, respectively. A
minimum contrast of 5 percent must be achieved for the films to be considered useful
as optical recording layers.
[0026] The thin amorphous film recording layers can be prepared by conventional thin film
deposition techniques such as evaporation, RF (radio frequency) and DC (direct current)
sputtering from an alloy target, and RF and DC co-sputtering from targets of the individual
elements. Enhancement of sputtering processes by applying magnetic fields (magnetron
sputtering) can also be used. The thickness of the films can be from a few tens to
a few hundreds nanometers depending on compromises among factors such as contrast,
sensitivity, production rate, material cost, ease of control, data rate, etc.
[0027] Supports which can be used include plastic films, such as polyethylene terephthalate,
polymethyl methacrylate, and polycarbonate, a glass plate, paper and metallic plates.
[0028] The practice of the invention is further described in the following examples. In
the examples, each thin film optical recording layer is represented by the symbol
Sb
xSn
yZn
z wherein x, y and z are atom percents.
Example 1
[0029] Two amorphous thin film optical recording layers of this invention were prepared
by a sputtering process. A target composed of mixed Sb and Zn powders was pre-sputtered
in an 8 mtorr Ar atmosphere for one hour. The pre-sputtering step was designed to
achieve a steady state deposition condition.
[0030] Thin films of about 80 nm in thickness were then prepared by sputtering the pre-sputtered
mix for 3.5 minutes. The sputtered mix was deposited as a thin film on a glass support.
The atomic fraction of each component in the prepared film was determined by inductively
coupled plasma atomic emission spectrometry (ICP).
[0031] Figure 3 shows the amorphous to crystalline transition temperature of thin films
of antimony-zinc comprising a) 11 atom percent zinc (curve 1) and b) 8 atom percent
zinc (curve 2). The transition temperatures were for film a) 156°C and for film b)
117°C. The heating rate was 25 milli-Kelvin per second. These high transition temperatures
show that the amorphous state of the films are stable. This is an important keeping
property. Spontaneous transition from amorphous to crystalline would be detrimental
to optical recording layers in that the reflectance difference between the crystalline
areas and amorphous areas would be lost.
[0032] Another sample of the thin antimony-zinc film comprising 8 atom percent of zinc was
written upon using the static pit tester described herein before. The writing was
in the form of crystallized marks on the films. The film (Sb₉₂ZN₈) with the crystallized
written spots was placed in a chamber at 70°C and 30 percent relative humidity for
an accelerated stability test. After 15 days, the film was examined. We did not observe
any phase change or corrosion on the unwritten area or the written spots. The film
did not have any overcoat as a protective layer against corrosion. This test shows
that the films of the invention bearing written spots are thermally and environmentally
stable.
[0033] Another film sample comprising 8 atom percent zinc was subjected to performance tests
on the static pit tester. A pulsed semiconductor laser beam with a wavelength of 830
nm was used for writing. The writing sensitivity and contract at various powers and
pulse widths are shown in Figure 4. The percent contrast between the initial reflectance
of the amorphous state and the final reflectance of the crystallized state is clearly
measurable and can thus be read by state of the art laser read systems. This data
also shows a) that the thin films can be written upon using practical laser powers
and writing speeds and b) the reflectivity of the crystalline state is higher than
the amorphous state.
Example 2
[0034] A number of amorphous Sb-Zn thin films with a range of compositions were prepared
according to the method in Example 1. Some representative compositions are Sb₉₄Zn₆,
Sb₈₆Zn₁₄ and Sb₈₂Zn₁₈. The first film can be written upon with a laser pulse length
of 50 ns and power of 6 mW. The second film can be written upon at a laser pulse length
of 100 ns and power of 6 mW. The last film can be written upon at a laser pulse length
of 1 µs and power of 4mW.
Example 3
[0035] THin films of about 100 nm in thickness were then prepared by sputtering as in example
1 but for 4 minutes. Figure 5 shows the amorphous to crystalline temperature and
reflectance of two different thin films of the invention.
[0036] The amorphous to crystalline transition temperature, the compositions and curve number
are set out below:
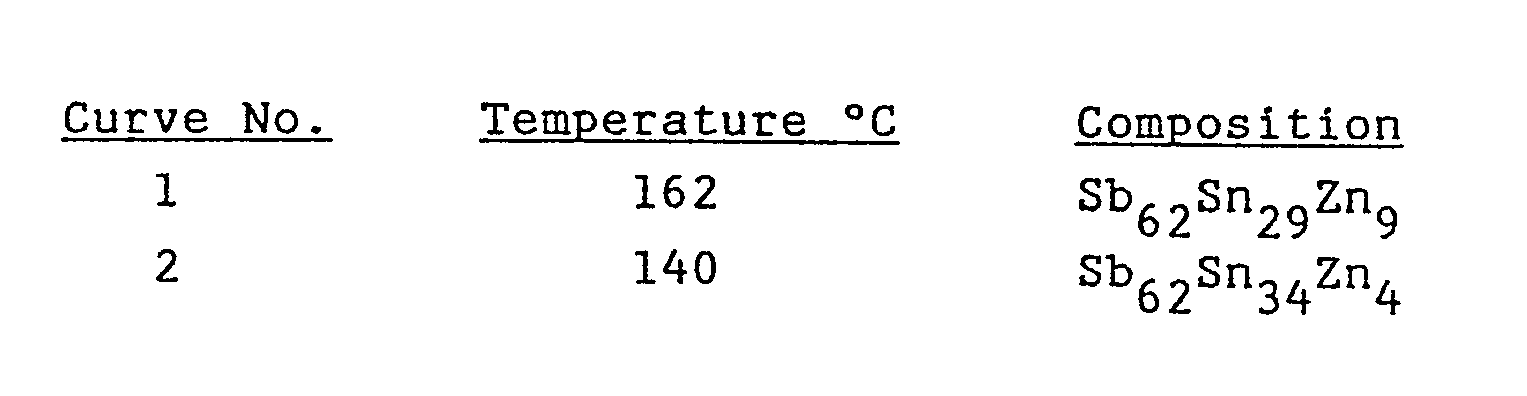
[0037] A comparison of Figure 3 to Figure 5 shows that SbZn films containing Sn have much
better contrast than SbZn films containing no Sn. The reflectance of the crystalline
areas were consistently greater than the amorphous areas.
[0038] Another thin film sample (Sb₆₂Sn₂₉Zn₉) was written upon using the static pit tester
described herein before. The writing was in the form of a pattern of amorphous and
crystallized marks on the films. The film with the crystallized written spots was
placed in a chamber at 70°C and 30 percent relative humidity for an accelerated stability
test. After 14 days, the film was examined. We did not observe any phase change or
corrosion on the unwritten film or the written spots. The film did not have any overcoat
as a protective layer against corrosion. This test shows that the films of the invention
bearing written spots are both thermally and environmentally stable.
[0039] Another film sample (Sb₆₂Sn₂₉Zn₉) was subjected to performance tests on the static
pit tester. A pulsed semiconductor laser beam with a wavelength of 830 nm was used
for writing. The writing sensitivity and contrast at various powers and pulse widths
are shown in Figure 6. Figure 6 shows the percent contrast between the initial reflectance
of the amorphous state and the final reflectance of the crystallized state is clearly
measurable and can thus be read by state of the art laser read systems. This data
also shows that the thin films can be written upon using practical laser powers and
writing speeds.
Example 4
[0040] A number of amorphous thin films with a range of compositions were prepared according
to the method in Example 1. Some of the representative compositions were Sb₅₉Sn₃₆Zn₅,
Sb₇₂Sn₈Zn₂₀, Sb₅₅Sn₂₅Zn₂₀, Sb₄₃Sn₂₄Zn₃₃, Sb₆₇Sn₁₉Zn₁₄, Sb₅₆Sn₂₂Zn₂₂. These films can
be written upon at a laser pulse length of 50 ns and power of 6mW.
Example 5
[0041] Several homogeneous Sb-Zn-Sn alloy sputtering targets with various compositions
were prepared by hot-pressing. The thin films were prepared by the sputtering process.
Some representative compositions are Sb₆₅Sn₂₀Zn₁₅, Sb₆₀Sn₂₈Zn₁₂, Sb₆₀Sn₂₀Zn₂₀ and
Sb₅₅Sn₂₀Zn₂₅. These films were amorphous and can be crystallized at a laser pulse
length of 50 ns and power of 4 mW.
[0042] None of the thin film optical recording layers in the above examples could be switched
between two different crystalline states.
Comparative Examples
[0043] Thin film layers were prepared in which the alloy compositions were a) Sb₄₀Sn₅₈Zn₂and
b) Sb₄₈Sn₂Zn₅₀. Film 1 was crystalline when deposited. Film 2 was amorphous when deposited,
but extremely difficult to crystallize. Both of these films are outside the scope
of the present invention.
1. A recording element comprising a write-once amorphous thin-film optical recording
layer of an alloy having a composition within a polygon in a ternary composition diagram
of antimony, zinc, and tin; wherein
a) the composition diagram is
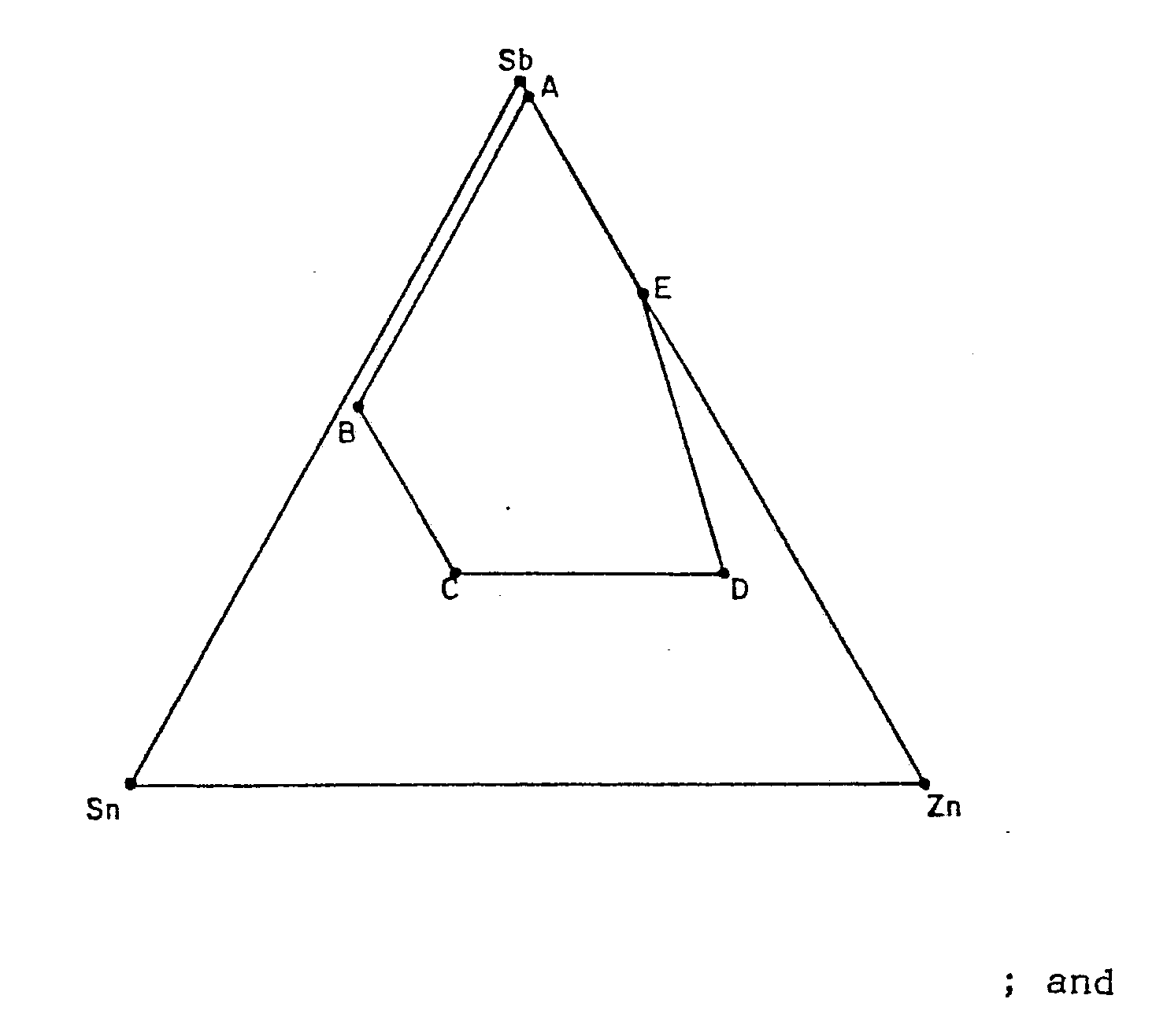
b) the polygon has the following vertices and corresponding coordinates in atom percent:
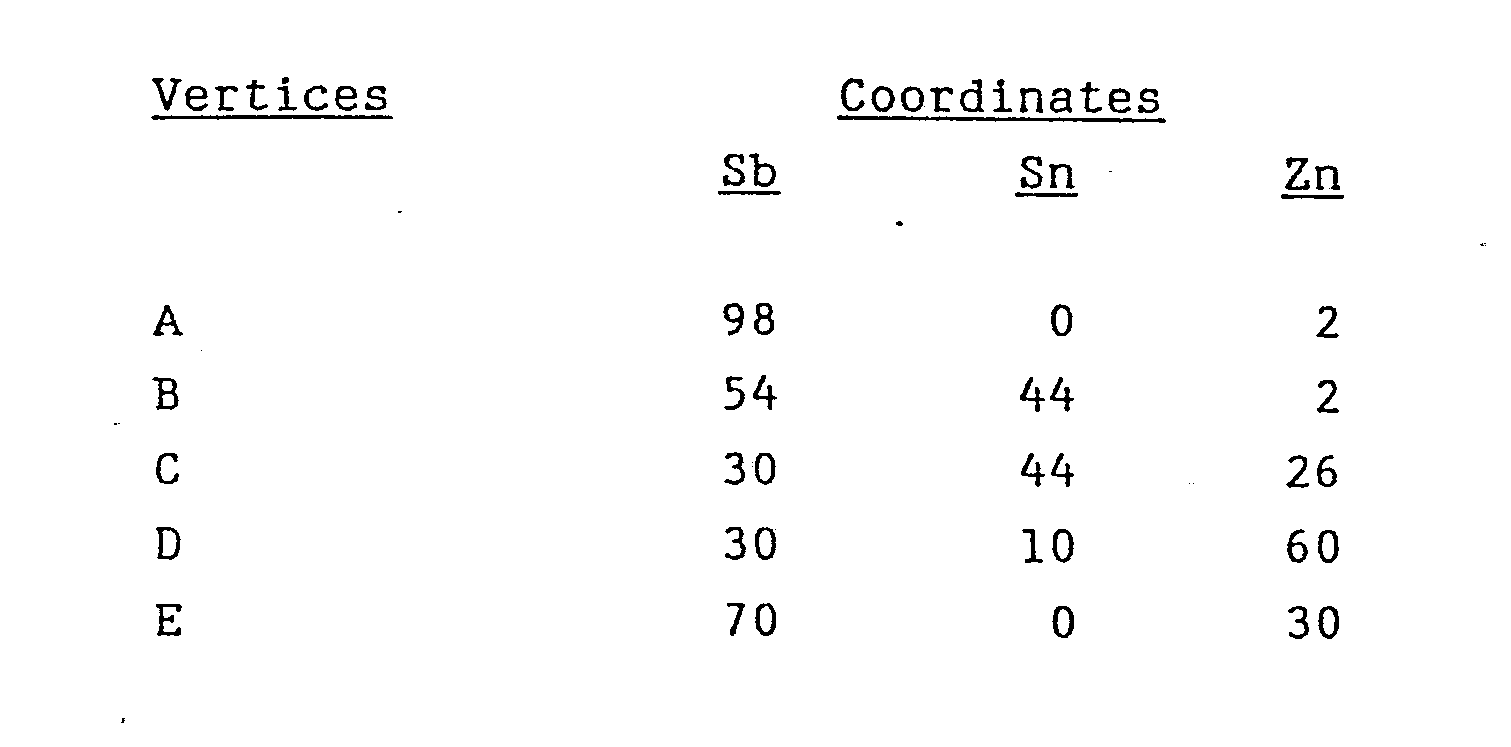
2. A record element comprising an optical record in a layer of an alloy having
a) a composition within a polygon in a ternary composition diagram of antimony, zinc,
and tin, wherein
i) the composition diagram is
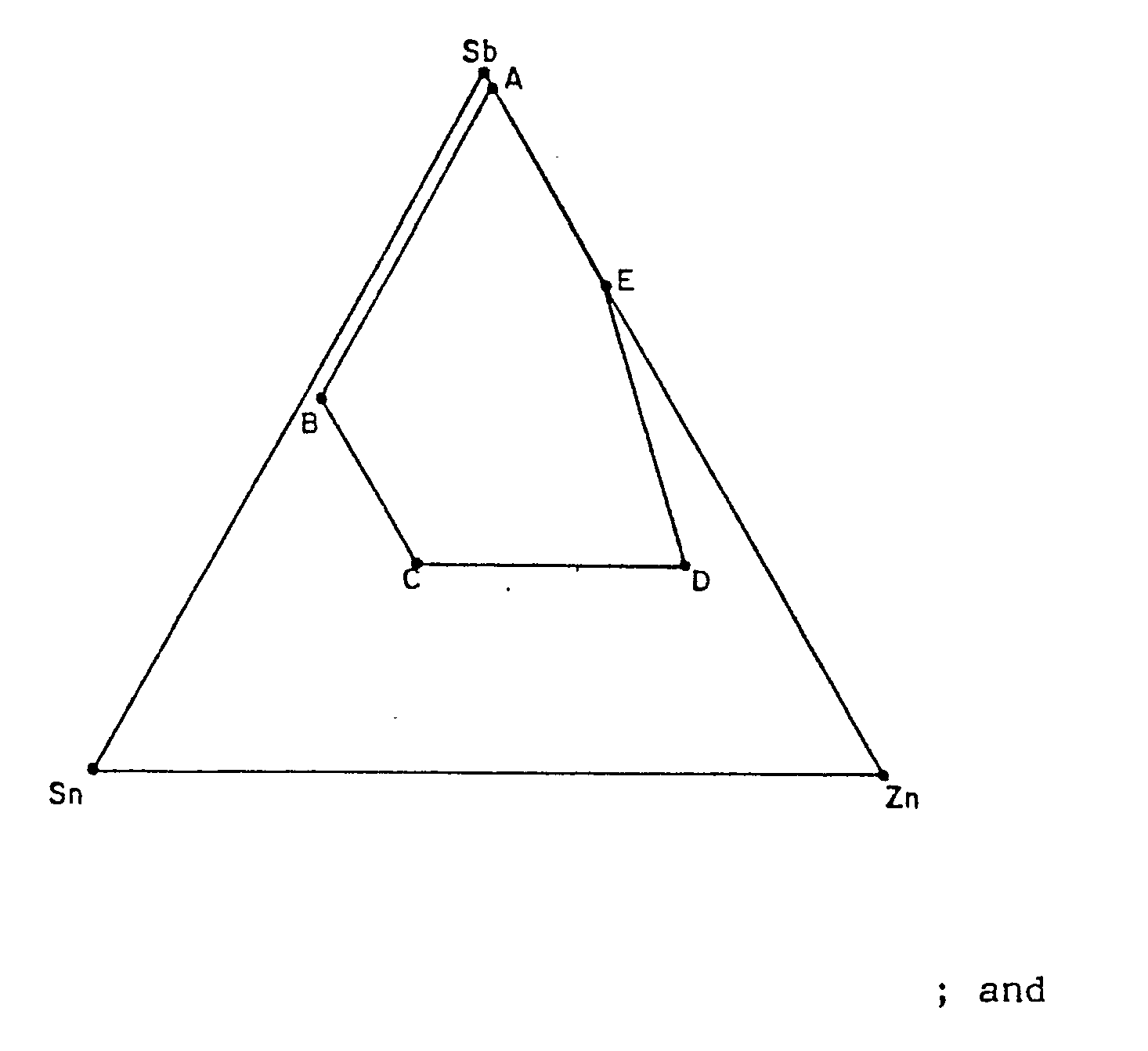
ii) the polygon has the following vertices and corresponding coordinates in
atom percent:

b) a pattern of amorphous and crystalline areas.
3. An element according to claim 1 or 2 wherein the alloy has a composition within
a polygon in the ternary phase-diagram
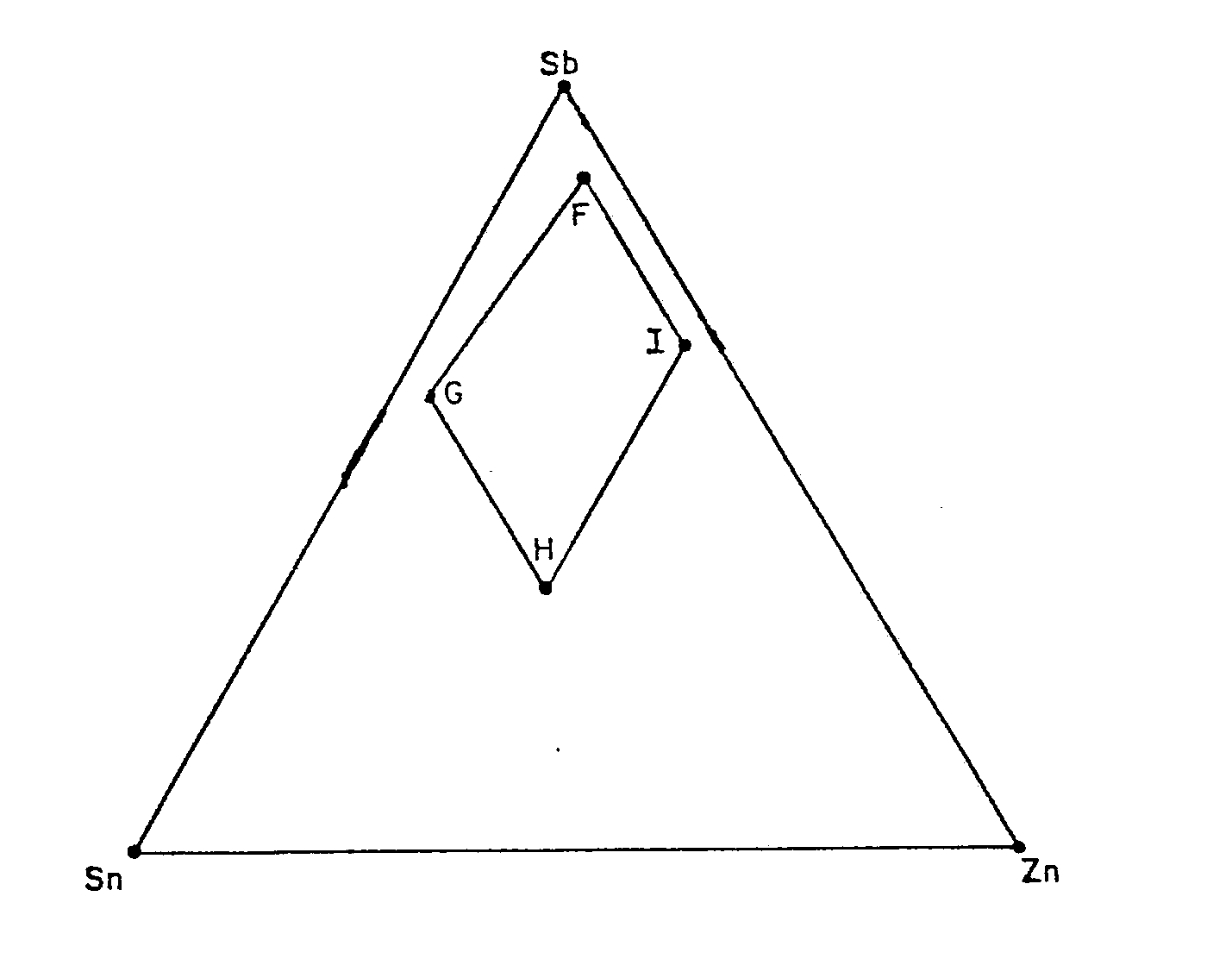
said composition being within the following vertices and corresponding coordinates:
4. The element of claim 1, 2 or 3 having an amorphous to crystalline transition temperature
of at least 80°C.
5. The element of claim 1 or 2 in which the layer is capable of exhibiting only a
single crystalline state having a substantially uniform composition.
6. The element of claim 1 or 2 in which the alloy has the composition Sb₆₅Sn₂₀Zn₁₅;
Sb₆₀Sn₂₈Zn₁₂; Sb₆₀Sn₂₀Zn₂₀ or Sb₅₅Sn₂₀Zn₂₅.
7. A method of recording information, comprising the steps of:
a) providing a recording element according to claim 1, 3, 4, 5 or 6; and
b) focusing an information modulated laser beam on the recording layer to form a pattern
of crystalline and amorphous areas in the layer.