Background of the Invention
[0001] The present invention relates to nonlinear optical loop mirror devices, and is directed
more particularly to nonlinear optical loop mirror devices which include optical fibers
having dispersions which decrease monotonically along the length thereof.
[0002] Nonlinear optical loop mirrors (NOLMs) and related nonlinear amplifying loop mirrors
(NALMs) have developed into important building blocks which are widely used in the
switching, shaping and other processing of optical pulses. In such loop mirrors, optical
pulses are coupled into a loop of optical fiber through a coupler that divides them
into two components pulses which propagate around the loop in opposite directions,
and which are transmitted and are reflected by the loop mirror, depending upon the
phases with which the component pulses return to the coupler. A NOLM of this general
type is described in "Nonlinear Optical Loop Mirror", by N. Doran and D. Wood, Optical
Letters, Vol. 13, No. 1, pp. 56-58 January 1988. A NALM of this general type is described
in "Nonlinear Optical Loop Mirror", by M. Fermann, et al., Optics Letters, Vol. 15,
No. 13, pp 752-754, July 1990
[0003] If a coupler divides an input pulse into two equal components pulses, and if the
loop affects these component pulses in the same way, i.e., symmetrically, the component
pulses will interfere constructively on their return to the coupler and, consequently,
will be reflected back through the coupler port through which they entered. If the
pulses are divided into unequal component pulses, and/or if the loop affects the component
pulses differently i.e., unsymmetrically or asymmetrically, the pulses may interfere
wither constructively, destructively or partly constructively and partly destructively.
In such cases the pulses return to the coupler may be reflected, transmitter or partly
reflected and partly transmitted. Since NOLMs which have asymmetrical properties provide
greater opportunities for useful signal processing, asymmetrical NOLMs are used much
more often than symmetrical NOLMs.
[0004] Asymmetrical NOLMs differs from one another primarily in the methods or structures
that are used to make them asymmetrical. One approach to introducing asymmetry into
a loop is to couple an input pulse into the loop with a power-coupling ratio that
differs from 50:50. One example of a NOLM that uses this form of asymmetry is described
in the above cited Doran and Wood articles.
[0005] Other approaches to introducing as asymmetry into a loop include locating rotated
sections of birefringent fiber therein, or positioning an optical amplifier asymmetrically
therein, An example of the form approach is described in "Optical Switching Using
Fiber Ring Reflectors", J. Mores, et al., J. Opt. Soc. Am B Vol. 8, No. 3, pp. 594-601,
March 1991. An example of the latter approach is described in the above-cited Fernann
et al., article.
[0006] Another known building block of optical fiber systems includes optical fibers that
have dispersions that vary along the length thereof. When the rate of change of this
dispersion is such as to balance the amplitude loss and pluses broadening that result
from transmission along a fiber, an optical pluses may be transmitted through the
fiber without its amplitude or temporal width being changed. Pulses which propagate
in this way are known as optical solitons or simply solitons. An example of an optical
fiber having such a variable dispersion is described in U.S. Pat. No. 4,962,987 (Doran)
and in "A Single-Mode Fiber with Chromatic Dispersion Varying Along the Length", V.
Bogatyryer, et al., Journal of Lightwave Technology, Vol 9, No. 5, pp. 561-566, May
1991.
[0007] Optical fibers having dispersion which decrease in the direction of propagation are
commonly referred to as dispersion decreasing (DD) fibers, while those having dispersions
which increase in the direction of propagation are commonly known as dispersion increasing
(Dl) fibers. As explained in the above-cited Doran patent and Bogatyrev article, such
fibers may be produced by changing the axial dopant concentration of the fiber, the
diameter of the fiber core or other fiber parameters.
[0008] Prior to the present invention, DD fibers were used to maintain the shape of optical
solitons in optical fiber waveguides having optical losses that were too large to
be neglected. This is because DD fibers allow a balance to be maintained between the
dispersion and nonlinear terms of the equation, commonly known as the nonlinear Schrodinger
equation, which governs the transmission of light pulse through optical fibers. DD
fibers have also found use as soliton pulses compressors and decompressors and in
devices which make use of the Raman scattering effect.
[0009] Prior to the present invention, however, dispersion DD and DI fibers have not been
used to introduced directional asymmetry into NOLMs and NALMs. As a result, NOLMs
and NALMs have not taken advantage of the many opportunities that DD and DI fibers
create for using NOLMs and NALMs to perform new optical functions or to perform known
optical functions in new and better ways.
[0010] Steele, in "Pulse compression by an optical fibre loop mirror constructed from two
different fibres", Electronics Letters, volume 29 (1993) pages 1972 to 1974, describes
a non-linear optical loop mirror which uses as the optical fiber of the loop a pair
of fibers with different optical properties that are spliced together.
SUMMARY OF THE INVENTION
[0011] In accordance with the present invention NOLM and NALM devices (hereinafter referred
to generically as loop mirror devices or loop mirrors) are combined with dispersion
decreasing fibers to produce new and powerful optical processing devices that may
be used to perform new optical processing functions or to perform known optical processing
function more efficiently and/or more cost effectively.
[0012] According to the invention, there is provided an optical apparatus as claimed in
claim 1.
[0013] Generally speaking, the present invention comprises a loop of optical fiber which
has a first end having a relatively high dispersion value and a second end having
a relatively low dispersion value, and which has a dispersion that decrease as a function
of the distance from it high dispersion end. The present invention also includes an
optical coupler, such as a interferometric coupler, having first and second loop ports
coupled to respective ends of the loop and having first and second I/O ports through
which optical pulses may be coupled into and out of that loop. Together, the coupler
and DD fiber loop constituted a loop mirror having a distribute directional asymmetry
that allows the loop mirror to be used in way that loop mirror with non-distributed
or lumped asymmetry structure cannot not, thereby making possible the performance
of new optical processing functions and the improved performance of known optical
processing functions.
[0014] In accordance with one important feature of the invention, the DD fiber has a dispersion
value which decreases monotonically from the high dispersion end of the loop to the
low dispersion end thereof. In the preferred embodiment, the change in dispersion
is distributed approximately continuously along the length of the loop, i.e., without
step like changes in either the diameter of the core of the fiber or the concentration
of dopant compounds. For many applications, however, it is acceptable to have the
change in dispersion distributed ova a plurality of discrete steps that are located
at predetermined intervals along the length of the fiber.
[0015] Advantageously, the dispersion of the fiber may be a function of distance, i.e.,
have a dispersion profile, which is specially optimized for particular loop mirror
applications. The axial dispersion profile may, for example, be selected so that a
predetermined relationship exists between the lengths of the steps and the soliton
periods or reaction lengths of the solitons with which the loop mirror is used. Further,
the number and sizes of the steps may be selected so that the dispersion profile of
the fiber is nonlinear, e.g. has a dispersion which decreases approximately exponentially.
Thus, the dispersion profile of the fiber used in the loop mirror of the invention
allows that loop mirror to produce a variety of asymmetrical effects that cannot be
produced with loop mirrors known prior to the present invention.
[0016] The use of a DD fiber in a loop mirror also provides the advantage that it presents
to the ports of its coupler both a first, high dispersion end and a second, low dispersion
end. Because to these differing dispersion values, optical pulses which are coupled
into the loop may comprise optical solitons of different orders, even when the coupler
provides a 50:50 coupling ratio. An input pulse may, for example, be divided into
a fundamental or first order soliton that propagates in the DD direction. Because
higher order solitons change shape or evolve in a periodic manner as they propagate
along a fiber, loop parameters such as the length, effective area, dispersion profile,
etc., of the fiber affect the ways in which solitons that have propagated around the
loop interact with one another on their return to the coupler. This, in turn, allows
the loop mirror to produce different effects on different types of input pulses, causing
some to interfere constructively and be reflected while causing others to interfere
destructively and be transmitted, depending upon input pulse parameters such as amplitude,
temporal width, etc. In other words, the above-mentioned variables among other, define
sets of loop parameters which allow the loop mirror of the invention to discriminate
between different types of input pulses based on differences in their pulse parameters.
[0017] Because of their ability to interact with and/or offset one another, the loop of
the loop mirror of the invention together define a multidimensional loop parameter
space from which may be selected combinations of loop parameters that have substantially
similar effects on input pulses. Because, for example, the point along a fiber at
which a higher order soliton recovers its original width and/or accumulates a 180
degree phase shift depends on both the dispersion profile and the effective area of
a fiber, this point may be made to occur at a particular point in a fiber by various
different combinations of dispersion profile and effective area. Similarly, other
combinations of loop parameters, such as initial and final dispersion values, rate
of change of dispersion, attenuation, etc. may be used to produce a similar effect
at the same or some other point in the fiber. It will therefore be understood that,
while the present description discloses particular advantageous combinations of loop
mirror parameters, these combinations are exemplary only, and are equivalent to other
combinations which produce substantially similar results in substantially similar
ways.
[0018] In one particularly important embodiment of the invention, the loop parameters are
selected so that the loop mirror may be used to distinguish between and separate optical
pulses on the basis of whether the temporal widths of those pulses are greater than
or less than a width. In this embodiment, which will be referred to as the pulse width
searching (PWS) embodiment, a 50:50 coupler is used to divide input pulses into two
counterpropagating component pulses with equal energies. The loop length and rate
of change of dispersion are selected so that the loop has differing effects on these
components pulses depending upon whether the input pulse from which they were derived
had a temporal width greater or less than the predetermined temporal width.
[0019] More particularly, the loop length and rate of change of dispersion are selected
so that relatively broad pulses have reaction lengths so long that they can respond
only to the average value of the dispersion around the loop, and consequently, cannot
form solitons of different orders. As a result, the two pulses are affected in the
same way by their propagation around the loop and do not return to the coupler with
a direction phase difference. Under these conditions, the returning pulses are approximately
in phase and are therefor reflected by the loop mirror. Relatively narrow pulses,
on the other hand, have reaction lengths short enough that they can respond to the
instantaneous value of the dispersion around the loop and are able to form solitons
of different orders. As a result, provided that their amplitudes are high enough,
the two pulses are affected differently by their propagation around the loop and return
to the coupler with a directional phase difference of approximately 180 degrees (pi
radians). Under these conditions, the returning pulses will be transmitted rather
than reflected by the loop mirror.
[0020] One application for the pulse width switching embodiment of the invention includes
loop mirrors which are able to separate high and low data rate channels that use the
same fiber. Another application is a pulse sorter that separates pulses that require
dispersion compensation from those that do not, thereby providing dispersion only
to pulses which require such compensation.
[0021] In another important embodiment of the invention the loop parameters are selected
so that the loop mirror of the invention may be used to distinguish between and separate
optical pulses on the basis of whether these pulses have amplitudes which are greater
or less than a predetermined amplitude. In this embodiment of the invention, which
will be referred to as the pulse amplitude switching (PAS) embodiment, a 50:50 coupler
is again used to divided input pulses into two counterpropagating component pulses.
In this case, how ever, the loop parameters are selected so that solitons of different
orders are formed only if the input pulses have an amplitude greater than a predetermined
minimum amplitude.
[0022] More particularly, for input pulses having amplitudes greater than the predetermined
minimum, solitons of different orders form and are able to accumulate a 180 degrees
phase difference upon returning to the coupler, provided that they do not have temporal
widths that are too great. Under this condition, the loop mirror of the invention
transmits the input pulse. For input pulses having amplitude, less than the predetermined
minimum, however, different order solitons are not produced. As a result, the counterpropagating
pulses are not able to accumulate any substantial phase difference as a result of
their propagation around the loop. Under these conditions, the loop mirror of the
invention reflects the input pulse.
[0023] One application for the pulse amplitude switching embodiment of the invention includes
a noise filter which separates high amplitude data pulses from low amplitude noise
pulses and thereby effectively increases the signal to noise ratio of an optical system.
DESCRIPTION OF THE DRAWINGS
[0024] Other objects and advantages of the invention will be apparent from the following
description and drawings, in which:
FIG. 1 show a non linear optical loop mirror constructed in accordance with the present
invention;
FIGS 2A and 2B are graphs showing the dispersion, as a function of distance, for two
dispersion decreasing fibers suitable for use in the loop mirror of FIGS. 1;
FIG 3 is a table showing equations which govern the operation of the loop mirror of
the invention;
FIG. 4 is a propagation diagram which shows the counter propagation of solitons of
different orders around the loop mirror of FIG> 1 and
FIGS. 5,6, and 7 illustrate specific applications of the loop mirror of the invention.
DESCRIPTION OF THE PREFERRED EMBODIMENTS
[0025] Referring to FIG. 1 there is shown a nonlinear optical loop mirror (NOLMs)10 which
has been constructed in accordance with the present invention. Loop mirror 10 includes
an optical fiber 20 fiber 20 having a first end 20-1 and a second end 20-2. Loop mirror
10 also includes an optical coupler 30 having a first pair of I/O ports 30-1 and 30-2
and a second pair of 30-3 and 30-4. When fiber 20 and coupler 30 are connected as
loop mirror, fiber 20 is formed into a loop 25 whose ends 20-1 and 20-2 are connected
to coupler I/O ports 30-3 and 30-4, respectively. Remaining I/O ports 30/1 and 30/2
are connected to the external devices of fibers with which loop mirror 10 is to used.
Because of their connection to the ends of loop 25 I/O ports 30-3 and 30-4 will hereinafter
be referred to loop ports.
[0026] Coupler 30 is preferably a coupler of the interferometric type, such as a beam splitter,
which serves couple optical pulses bidirectionally between 1/0 ports 30-1 and 30-2
and loop 25. More particularly, coupler 30 serves to divide optical pulses entering
either of I/O ports 30-1 and 30-2 into two component optical pulses which propagate
around loop 25 in opposite directions, i.e., counterpropagate. Coupler 30 also serves
to receive returning components pulses and to divide the energy thereof between I/O
ports 30-1 and 30-2 in accordance with the relative amplitudes, widths and phases
thereof. To the extent that returning pulses are directed out of the same I/O through
which they entered, they are said to be "reflected" or "unswitched"; to the extent
that returning pulses are directed out of the other I/O port, they are said be to
"transmitted" or "switched". Because coupler 30 is of a type that is known to those
skilled in the art, it will not be described in detail herein.
[0027] In accordance with the present invention, fiber 20 comprises a fiber having a dispersion
D (often referred to a group velocity dispersion or GVD) that varies as a predetermined
function of the distance Z from one of the ends thereof. In the preferred embodiment,
dispersion D has a maximum value D
max at end 20-1 of fiber 20 and a minimum value D
min at end 20-2 thereof and has a magnitude that deceases monotonically as a function
of the distance from end 20-1. Accordingly, it will be seen that fiber 20 comprises
decreasing (DD) fiber for optical pulses that propagated around loop 25 in the clockwise
or DD direction, as shown in FIG. 1 and to comprise a dispersion increasing (DI) fiber
for optical pulses that propagate around loop 25 in the counterclockwise or DI direction,
as shown in FIG 1. As a result, fiber 20 of the invention introduces into the loop
mirror of FIG. 1 a directional asymmetry that cause counterpropagating optical pulses
to be affected differently by their propagate around loop 25. As will be explained
more fully later, this directional asymmetry allows the loop mirror of the invention
not only to perform optical functions that have previously been preformed with other
asymmetry introducing structures, such as birefringent fibers, but to perform those
functions better.
[0028] In the preferred embodiment, the dispersion of fiber 20 decreases exponentially as
a function of distance z approximately in accordance with equation 1 of FIG. 3. When
plotted as a function of distance, dispersion D has the general appearance shown in
FIGS. 2A and 2B in the case of FIG. 2A, dispersion D decreases monotonically in a
plurality of discrete steps which comprise segments of fiber having predetermined
respective lengths and predetermined approximately constant respective dispersions.
In the case of FIG. 2B, the dispersion decreases monotonically in an approximately
continuous manner. In both cases, however, the decrease in dispersion is distributed
along substantially the entire length of loop 25. As a result, pulses propagated around
loop 25 are exposed to a variable dispersion gradient or dispersion profile that extends
from one end of the loop 25 to the other.
[0029] A fiber having the variable dispersion profile shown in FIG. 2A may be produced in
a variety of ways. One of these includes the drawing of the fiber from a specially
designed preform in such a way that the fiber has a constant outside diameter, but
a core area that decrease in the FF direction. Another of these includes the drawing
of the fiber from a specially designed preform that is constructed so that the fiber
has a dopant t level and, consequently, an index of refraction that varies as a function
of distance along the length thereof.
[0030] Among fibers that have dispersion profiles such as those shown in FIGS. 2A and 2B,
there are many possible combinations of loop parameters which influence the way that
the loop affects optical pulses propagating thereto. Included among these loop parameters
are: the length of the fiber, the attenuation of the fiber, the dispersion values
at the ends of the fiber; the mathematical form and coefficients of the dispersion
function, the number and lengths of the segments making up the steps in (in stepped
embodiments such as that shown in FIG. 2A), the effective area of the fiber, and the
cross-sectional area of the core of the fiber. Of the last mentioned areas, the core
area is simply the geometrical cross-sectional area of the core portion of the fiber.
The effective area of the fiber, on the other hand, takes into account not only the
geometrical area of the core but also the nonlinearity of the fiber. Together with
the nonlinear index of refraction N2 of the fiber, the effective area of the fiber
determines the nonlinear coefficient of the fiber, a quantity shown as G in equation
2 of FIG. 3
[0031] Depending upon whether or not coupler 30 is regarded as a part of the loop, the loop
parameters may be regarded as including or not including the coupling ratio of coupler
30. Because the remaining loop parameters themselves provided any necessary directional
asymmetry, it will not ordinarily be necessary to use coupling ratios other than 50:50
coupling ratio for this purpose. As a result, the present invention will ordinarily
use a 50:50 coupling ratio, (thereby effectively eliminating the coupling ratio as
a loop parameter), although other coupling ratios may be used, if desired.
[0032] In addition, there are a number of possible combinations of temporal and spectral
pulse parameters which influence the way that a pulse is affected by particular combinations
of loop parameters. Included among the temporal parameters of a pulse are its amplitude
or peak powers, its order (for solitons), and its temporal width, the latter often
being referred to "full width half magnitude" or "FWHM". Included among the spectral
parameters of a pulses are its central wavelength, its spectral width, and its chirp.
[0033] In accordance with the present invention, it has been discovered that particular
combinations of loop parameters can be used to distinguish optical pulses having one
set of pulse parameters from those having another set of pulse parameters. This ability
to distinguish between pulses with different pulse parameters, in turn, allows the
loop mirror of the invention to separate, sort or route pulses based on those differences.
Once this has been done, the separated pulses may be further differentially processed
by, for example, amplifying one and not the other, compressing one and not the other,
etc. Thus, the loop mirror of the invention comprises a powerful general purpose optical
processing device that may be used in a variety of different applications.
[0034] In a first, pulse width switching embodiment the loop mirror of the invention is
used to separate pulses on the basis of differences in the temporal widths thereof,
provided that their amplitudes are great enough to be in the soliton regime. More
particularly, the loop mirror is used to reflect (or not switch) input pulses which
have temporal widths that are greater than a predetermined minimum width and to transmit
(or switch) at least a substantial fraction of the energy of input pulses which have
temporal widths that are less than that minimum width. The manner in which this is
accomplished will now be described with reference to FIGS. 1 and 4.
[0035] In configuring the loop mirror of FIG. 1 for use as pulse width dependent switch,
its loop parameters are selected on the basis of the pulse parameters that are to
be switched. This selection may be summarized as follows: The length of the loop and
the number and lengths of the dispersion steps are selected so that pulses having
widths greater than the minimum width (wide pulses) have a reaction length which is
long in relation to the lengths of the steps, but so that pulses having less than
the minimum width (narrow pulses) have a reaction length which is short in relation
to the lengths of the steps. For pulses having amplitudes that are great enough to
be in the soliton regime, this is comparable to saying that wide pulses have solitons
periods Z
0 which are long in relation to the length of the loop, while narrow pulses do not.
[0036] Assuming, for example, that the minimum pulse width is 7.5 picoseconds (ps), the
loop may have a length of 8.8 km with a dispersion that decreases from -9 to -1 ps
2/km in 8 steps, with each step comprising a segment of fiber having a length of 1
km and a dispersion which is approximately constant along the length thereof. With
such a pulse and such a loop it has been found that the percentage of the energy of
a pulse entering 1/0 port 30-1 which is transmitted to I/O port 30-2 falls substantially
as the width of the input pulse rises above 7.5 ps, falling to less than 8% as the
pulse width approaches 9 ps. Conversely, it has been found that the percentage of
the input energy which is transmitted increases substantially as the width of the
input pulse falls below 7.5 ps, rising to more that 50% as the pulse width approaches
5 ps. Pulses which have which have amplitudes that are to low to be in the soliton
regime (i.e., are in the linear regime) are reflected without regard to their widths.
For reasons which will be described more fully later, the just described combination
of loop parameters is only an exemplary one of a number of possible combinations of
loop parameters which will produce the just described result.
[0037] The reasons why the loop mirror of the invention produces the above-described pulse
width switching effect will now be described. Given the above-described relationships
of segment length and temporal width, relatively wide pulses, such as those with temporal
widths greater than 7.5ps, have a reaction length which is too great to allow then
to react to the individual steps of the DD fiber. This assures that, when coupler
30 divides an input pulse at I/O port 30-1 into two counterpropagating component pulses,
the two component pulses both react to the average of the dispersion values of the
steps, rather than to the individual dispersion values thereof. This, in turn, causes
the counterpropagation component pulses to be similarly affected by their propagation
around the loop. As a result, the two broad component pulses return to the coupler
with similar amplitudes and widths and in an approximately in-phase relationship with
respect one another and, consequently, are reflected.
[0038] As relatively narrow input pulses are applied to I/O port 30-1, however, their shorter
reaction length allows then to respond to the individual steps of the DD fiber. Then,
provided that an input pulse has an amplitude in the soliton regime, it will be divided
into two counterpropagating solitons of different orders which are affected differently
by their propagation around the loop. In accordance with the present invention, a
suitable combination of loop parameters causes the returning different order solitons
to return to coupler 30 with similar amplitudes and widths but in a generally out-of-phase
relationship (e.g., with a phase difference on the order of 180 degrees or pi radians).
As a result, the two returning solitons tend to be transmitted rather than reflected.
[0039] The above-mentioned result is best understood with reference to equation (2) of FIG.
3. The latter equation shows the relationship between the order number N of soliton,
the peak power P
0 and temporal width T
0 thereof and the local dispersion D of the fiber in which the soliton is propagating.
This equation show that, when a pulse having combination of amplitude and width within
the soliton regime is split into two component pulses with equal energies and applied
to a fiber having ends with differing dispersions, the result can be a pair of counterpropagating
component solitons which have different order numbers, the difference in order depending
on the difference in the dispersion values seen by the component pulses. If different
order solitons are generated, they will, initially at least, have equal amplitudes
with equal widths. During propagation, however, higher order solitons will undergo
change in their shape, periodically recovering their original shape at distances related
to their soliton periods, while first order solitons do not undergo such a periodic
change in shape. This difference in evolution is illustrated in FIG. 4.
[0040] In the case of pulses which are relatively broad, both component pulses "see" a loop
having the same dispersion value. This is because their reaction lengths are too great
to be effected by the individual dispersion steps and, consequently, both component
pulses see a loop having a dispersion equal to the average of the dispersion of the
steps. Under these conditions, no different order solitons are formed. As a result,
the component pulses are affected in the same way by their propagation around the
loop and, consequently return to coupler 30 in a generally in phase relationship.
Under these conditions, coupler 30 directs most of the returning energy to I/O port
30-1 and the input pulse may be said to be reflected.
[0041] In the case of pulses which are relatively narrow, on the other hand, the component
pulses "see" loops having different dispersion. This is because their shorter reaction
lengths allow them to be affected by the individual dispersions steps, allowing one
component pulse to see D
max while the other sees D
min.. As a result the input pulse is divided into two component solitons which have equal
energies but different orders. One of these may for example be a first order soliton
which propagates in DD or CW direction and undergoes no substantial change in amplitude
or width. The other may be a third order soliton which propagates in DI or CCW direction
and spends much of its propagation time with an amplitude greater than that with which
it entered the loop and with a width that varies as a periodic function of distance.
This periodically varying amplitude and width may be seen in FIG. 4.
[0042] Because the third order soliton spends a substantial fraction of its propagation
time with a higher amplitude than the first order soliton, it is more affected by
the non-linear optical properties of the fibers than the first order soliton. As a
result, it undergoes a greater degree of self phase modulation than the first order
soliton. This, in turn, causes the first and third order solitons to return to the
coupler in a generally out of phase relationship. For an appropriate choice of loop
length and rate of change of dispersion, the difference in the phase of these solitons
may be made approximately equal to 180 degrees while the temporal width thereof returns
to the equal widths with which they entered the loop. To the extent that these condition
are met, the energy of the input pulse will be transmitted through coupler I/O port
30-2, and the input pulse may be said to be transmitted.
[0043] In view of the foregoing, it will be seen that the above-described embodiment of
the invention will controllably reflect or transmit input pulses, base on differences
in their temporal width, provided that their amplitudes are within the soliton regime.
[0044] Referring to FIG. 5, there is shown one example of a specific application of the
pulse width switching embodiment of the loop mirror of the invention, namely: a noise
filter for filtering amplified spontaneous emissions (ASE) noise. The loop mirror
of FIG. 5 will be understood to be generally similar to that shown in FIG. 1, like
functioning parts being similarly numbered, but to have a combination of loop parameters
which allows it to differentiate between pulses of the basis of differences in their
widths.
[0045] In FIG. 5, the input of loop mirror 10 comprises an optical signal OS1 that includes
a relatively narrow data pulse D1 and an ASE noise component N1 that may be visualized
as a relatively wide pulse. Provided that the form has temporal width that is less
than the minimum width at which loop mirror 10 transmits input pulses, and that the
latter has a width greater than that minimum width, these two components will become
separated as a result of their encounter with loop mirror 10. More particularly, data
pulse D1 will be largely transmitted by loop mirror 10 and will exit coupler 30 at
I/O port 30-2 as a pulse D1
1 from which most ASE noise has been filtered. At the same time, noise "pulse" N1 will
be largely reflected by loop mirror 10 and will exit coupler 30 at I/O port 30-1 as
a noise pulse N1
1 from which most of the data pulse has been removed. Because the separating action
of loop mirror 10 also requires that the pulse to be transmitted have an amplitude
in the soliton regime, the loop mirror of FIG. 5 also tends to reflect and thereby
remove from the transmitted signal at I/O port 30-2 low amplitude noise pulses which
may have unintentionally become entrained in input signal OSI. Thus, the loop mirror
of FIG. 5 will be seen to act as a filter for both ASE noise and low amplitude pulse
noise.
[0046] Referring to FIG. 6, there is shown another exemplary application of the pulse width
switching embodiment of the loop mirror of the invention. In the application shown
in FIG. 6, the loop mirror serves as a return to zero (RZ) demultiplexer which separates
two data channels made up of pulse trains that include pulses with different widths
and different repetition rates.
[0047] In FIG. 6, the input of loop mirror 10 comprises an optical signal OS2 that includes
a first data channel which takes the form of a pulse train OS2A made up of pulses
that are relatively wide and have a relatively low repetition rate and a second data
channel which takes the form of a pulse train OS2B made up of pulses that are relatively
narrow and have a relatively high repetition rate. Upon entering loop mirror 10 the
pulses making up these two channels will be separated on the basis of their widths,
provided that the pulses of the pulse train to be transmitted have amplitudes that
are in the soliton regime. More particularly, the relatively broad pulses of input
pulse train OS2A will propagate around the loop and be reflected out of coupler I/O
port 30-1, while the relatively narrow pulses of input pulse train OS2B will propagate
around the loop and be transmitted out of coupler I/O port 30-2. Because the manner
in which this separation takes place has already been described, the embodiment of
FIG. 6 will not be further discussed herein.
[0048] In a second, pulse amplitude switching embodiment, the ability of the loop mirror
of the invention to discriminate between pulses on the basis of their pulse parameters
is used to separate pulses on the basis of differences in the amplitudes or peak powers
thereof. More particularly, the loop mirror is used to reflect input pulses having
amplitudes that are less than a predetermined value and to transmit pulses having
amplitudes that are greater than that predetermined value, provided that their widths
are not so great that the above-described pulse width switching effect comes into
play.
[0049] To the end that the pulse amplitude switching embodiment may separate pulses on the
basis of differences in their amplitudes, the parameters of loop 25 are selected so
that an input pulse having an amplitude less than the minimum value (low intensity
or dim pulses) is divided into component pulses that are not solitons of different
orders or even solitons of the same order. Because such pulses are affected in the
same way by their propagation around the loop, they return to the coupler in a generally
in-phase relationship. Under these conditions, the input pulse will be routed to I/O
port 30-1, i.e., will be reflected rather than transmitted.
[0050] The parameters of the loop are also selected so that input pulses having amplitudes
greater than the minimum value (high intensity or bright pulses) are divided into
component pulses which are solitons of different orders, and so that the component
solitons return to the coupler in a generally out-of-phase relationship, but with
similar amplitudes and temporal widths. To the extent that these conditions are met,
the input pulse will be routed to I/O port 30-2, i.e., will be transmitted rather
than reflected.
[0051] In achieving the above-described result, the parameters of the loop are selected
to be somewhat different from those of the previously described pulse width switching
embodiment. In particular, the dispersion of the fiber is selected so that the loop
has a lower rate of change of dispersion. The assures that component pulses which
are not solitons are not affected in substantially different ways by their propagation
around the loop. In addition, other loop parameters such as the length of the loop,
and the effective area of the fiber are selected in relation to the rate of change
of the dispersion of the fiber so that, for component pulses which are solitons of
different orders, the component pulses return to the coupler with equal widths. Because
of their initially equal energies and equal propagation losses, such component pulses
also return to the coupler with equal amplitudes. This amplitude and width matching
assures high contrast switching provided that the returning pulses also interfere
destructively on their return to the coupler. As explained earlier in connection with
the pulse width switching embodiment, this out-of-phase relationship is established
by taking advantage of the fact that the higher order soliton spends a higher proportion
of its propagation time with a peak value that exceeds that of the first order soliton
and, consequently, undergoes a different phase shift as a result of self phase modulation.
Because this effect has already been described in connection with FIG. 4 and the pulse
width switching embodiment, it will not be further discussed herein.
[0052] Referring to FIG. 7 there is shown one example of a specific application of the pulse
amplitude switching embodiment of the loop mirror of the invention, namely: a noise
filter for filtering noise "pulses" from a train of data pulses. The loop mirror of
FIG. 7 is generally similar to that shown in FIG. 1, but has loop parameters which
are selected to cause it to differentiate pulses on the basis of differences in their
amplitudes, i.e., their peak powers or intensities.
[0053] In FIG. 7 the input signal of the loop mirror comprises an optical signal OS3 that
includes a data pulse D3 having an amplitude A3 that exceeds the minimum amplitude
A
MIN at which pulses may be transmitted by the loop mirror. (This minimum amplitude may
be regarded as the threshold which separates combinations of amplitude and pulse width
which separate the celation regime from the linear regime; for the sake of clarity
all illustrated pulses are shown as having similar temporal widths). Optical signal
OS3 also includes two noise pulse N2 and N3 which have amplitudes that are less than
A
MIN. Such pulses may, for example, comprise non data bearing pulses which are the result
from unintended cross coupling between fibers or the result of amplification effects.
[0054] Because data pulse D3 has an amplitude that exceeds the amplitude minimum for the
loop mirror, it will be split into component solitons of different orders which return
to coupler 30 in a generally out of phase relationship as a result of their differing
temporal evolutions, as shown in FIG. 4. This, together, with similarities in the
amplitudes and widths of the returning component solitons, assures that pulse D3 is
transmitted I/O port 30-2. Noise pulses N2 and N3, however, are not split into such
component solitons. As a result, the component pulses propagate around the loop without
accumulating any substantial difference in phase and, consequently, are reflected
through I/O port 30-1. Thus, the loop mirror of FIG. 7 serves as a noise filter which
allows low amplitude noise pulses to be efficiently separated from high amplitude
data pulses.
[0055] In view of the foregoing it will be seen that, in spite of the different uses to
which they are put, the pulse amplitude and pulse width switching embodiments of the
loop mirror of the invention make use of substantially the same direction dependent
phase shifting effect. Stated differently, the pulse amplitude and pulse width switching
embodiments of the invention accomplish different end results because they use the
same direction dependent phase shifting effect in conjunction with different combinations
of loop and pulse parameters.
[0056] In selecting the combinations of loop and pulse parameters that are used in practicing
the present invention, care must be exercised to take into account the interactions
and potential tradeoff between the various loop parameters. In the case of the pulse
amplitude switching embodiment, for example, the length of the fiber and the effective
area thereof interact so that the two can be traded off against one another in ways
that allow the higher order soliton to recover its original width on returning to
the coupler. Both of these parameters, in turn, are affected by the dispersion values
at the first and second ends of the fiber and the mathematical form and coefficients
of the dispersion as a function of distance along the fiber. It will, therefore, in
general be necessary to calculate a set of loop parameters on the basis of a set of
simultaneous equations which together define all of the requirements to be met. Because
computer programs (including numerical approximation programs) suitable for use in
solving such sets of simultaneous equations are known to those skilled in the art,
such solitons will not be discussed in detail herein.
[0057] In addition, the calculation of a set of loop parameters must take into account the
dynamic changes that occur in the pulse parameters as a pulse propagates around the
loop. Referring to equation (2) of FIG. 3, for example, it will be seen that, as a
soliton pulse encounters a sequence of different local dispersion values during propagation,
both its soliton period and its order number can change. A solition that begins propagating
as a third order soliton may therefore be transformed into first order soliton before
it returns to the coupler.
[0058] Propagation dependent effects of the above-described type are particularly complex,
in cases in which both of the counterpropagating solutions are higher order solitons.
This is because, in such cases, both of the solitons have waveforms that vary as periodic
functions of variable
z/
Z0 that indicates the fractions or multiples of a soliton period that correspond to
a particular location within the loop. Accordingly, while the present invention in
its broadest aspect encompasses different order solitons of any combination of orders,
it preferably encompasses different order solutions in which one of the solitons is
a first order or fundamental soliton.
[0059] While this invention has been explained with reference to the structure disclosed
herein, it is not confined to the details set forth and this application is intended
to cover any modifications and changes as may come within the scope of the following
claims:
1. An optical fiber apparatus for use with optical pulses, comprising:
a) an optical fiber including a first end having a relatively high dispersion and
a second end having a relatively low dispersion,
b) an optical coupler having first and second I/O ports and first and second loop
ports, said first and second loop ports being connected to the first and second ends
of said fiber to form said fiber into a loop which is described by a set of loop parameters
comprising the length, the effective area, and the dispersion values at the first
and second ends of said fiber comprising said loop, and the mathematical form of said
monotonic function, which describes the dispersion of said fiber, said coupler serving
as means for;
i) dividing an input pulse which is described by a set of pulse parameters comprising
the amplitude and temporal width of said input pulse, entering one of said I/O ports
into component pulses which counter propagate around said loop, and
ii) receiving component pulses returning to said coupler after propagating around
said loop and dividing the energy of the returning component pulses between said first
and second I/O ports in accordance with the relative phases thereof;
c) characterised in that the optical fiber has a dispersion which decreases as a monotonic function of the
distance from said first end of said fiber;
d) said loop differentially affecting said counter propagating pulses so that said
loop parameters cause the energy of the received pulses to be divided between said
I/O ports in accordance with said pulse parameters.
2. An optical fiber apparatus as set forth in claim 1 in which said optical coupler provides
an approximately 50:50 coupling ratio.
3. An optical fiber apparatus as set forth in claim 1 in which the dispersion of said
fiber decreases approximately exponentially from said first end to said second end.
4. An optical fiber apparatus as set forth in claim 3 in which the dispersion D of said
fiber varies with the distance Z from the first end of said fiber approximately in
accordance with the equation:
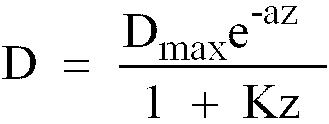
where D
MAX is the dispersion of said fiber at said first end, R is the exponential rate of change
of dispersion of said fiber and K is a constant.
5. An optical fiber apparatus as set forth in claim 1 in which the dispersion of said
fiber decreases in a plurality of steps from said first end to said second end, each
step comprising a fiber segment having a predetermined respective length and an approximately
constant respective dispersion.
6. An optical fiber apparatus as set forth in claim 1 in which the dispersion of said
fiber decreases approximately continuously from said first end to said second end.
7. An optical fiber apparatus as set forth in claim 1 in which said fiber has a core
portion having a first index of refraction and a diameter and a cladding portion having
a second index of refraction, and an outside diameter which is approximately constant,
wherein the diameter of said core portion decreases monotonically from said first
end to said second end.
8. An optical fiber apparatus as set forth in claim 7 in which the differences between
said first and second indexes of refraction result from differences between the concentrations
of dopants in said core portion and said cladding portion, and in which the dopant
level of at least one of said portions changes monotonically from said first end to
said second end.
9. An optical fiber apparatus as set forth in claim 1 in which the dispersions at the
first and second ends of said fiber have magnitudes such that input pulses having
suitable combinations of peak power and temporal width propagate in said loop as solitons.
10. An optical fiber apparatus as set forth in claim 5 in which the dispersion at the
first and second ends of said fiber have magnitudes such that input pluses having
suitable combinations of peak power and temporal width propagate in said loop as solitons.
11. An optical fiber apparatus as set forth in claim 10 in which the lengths of said segments
are small in relation to the local periods of said solitons.
12. An optical fiber apparatus as set forth in claim 10 in which the number of said segments
is large in relation to the local periods of said solitons.
13. An optical fiber apparatus as set forth in claim 1 in which said fiber has a dispersion
in the anomalous dispersion regime.
14. An optical fiber apparatus as set forth in claim 1 wherein, at least the dispersion
values at the first and second ends of said fiber comprising said loop, and the mathematical
form of said monotonic function, which describes the dispersion of said fiber are
chosen to provide a loop in which
a pulse, having a temporal width greater than a pre-selected width, has a phase
entering the loop essentially the same as the pulse phase after propagation through
the loop, and
a pulse, having a temporal width less than said pre-selected width, has a phase
entering the loop different from the pulse phase after propagation through the loop.
15. An optical fiber apparatus as set forth in claim 14 in which the widths of pluses
having temporal widths greater than said predetermined temporal width are large in
relation to the length of said loop.
16. An optical fiber apparatus as set forth in claim 14 in which input pulses having temporal
widths less than said predetermined temporal width have a reaction length which is
relatively short relation to the length of said loop, and in which input pulse having
temporal widths greater than said predetermined temporal width have a reaction length
which is relatively long in relation to the length of said loop.
17. An optical fiber apparatus as set forth in claim 14 in which said coupler divides
an input pulse having a temporal width less than said predetermined temporal width
in to a lower order component soliton and a higher order component soliton, said higher
order component soliton having an amplitude which exceeds that of said lower order
component soliton during a substantial part of the time that said solitons are propagating
around said loop.
18. An optical fiber apparatus as set forth in claim 17 in which said coupler divides
an input pulse having a temporal width greater than said predetermined temporal width
into solitons of the same order.
19. An optical fiber apparatus as set forth in claim 17 in which the dispersion of said
fiber decreases approximately continuously from said first end to said second end.
20. An optical fiber apparatus as set forth in claim 17 in which the dispersion of said
fiber changes in a plurality of steps, each step comprising a fiber segment having
a predetermined respective length and an approximately constant respective dispersion.
21. An optical fiber apparatus as set forth in claim 20 in which the lengths of said segments
are small in relation to the local periods of said solutions.
22. An optical fiber apparatus as set forth in claim 20 in which input pluses having temporal
widths greater than said predetermined temporal width respond substantially to the
average of the dispersion values of said steps, and input pulses having temporal widths
less than said predetermine temporal width respond to the individual dispersion values
of said steps.
23. An optical fiber apparatus as set forth in claim 20 in which the dispersion values
of said steps decrease exponentially from said first end to said second end.
24. An optical fiber apparatus as set forth in claim 14 in which said fiber has a dispersion
in the anomalous dispersion regime.
25. An optical fiber apparatus as set forth in claim 14 wherein said component pulses,
having a temporal width less than said pre-selected width, are solitons of the same
or different order.
26. An optical fiber apparatus as set forth in claim 25 in which said solitons include
a lower order soliton and a higher order soliton and in which said higher order soliton
has an amplitude which exceeds that of said lower order soliton during a substantial
part of the time that said solitons are propagating around said loop.
27. An optical fiber apparatus as set forth in claim 25 in which the dispersion values
of said steps decrease exponentially from said first end to said second end.
28. An optical fiber apparatus as set forth in claim 1 wherein said loop parameters further
include the attenuation and effective area of said fiber and the relationship among
said parameters including attenuation, effective area, the dispersion at said fiber
ends, and the dependence of dispersion on distance from the first said fiber end,
is such that,
pulses having an amplitude below a pre-selected amplitude are divided into component
pulses which are not solitons and which have a phase which remains substantially the
same before and after propagation around said loop, and
pulses having an amplitude above the pre-selected amplitude are divided into component
pulses which are solitons of different or the same order and which have a phase which
changes in propagation around said loop.
29. An optical fiber apparatus as set forth in claim 28 in which said different order
solitons include a lower order component soliton and a higher order component soliton,
in which said lower order component soliton returns to said coupler with a temporal
width approximately equal to that with which it entered said loop in which said higher
order component solitons returns to said coupler with a temporal width approximately
equal to that of said lower order soliton and in which the lower and higher order
soliton return to said coupler in a generally out-of-phase relationship.
30. An optical fiber apparatus as set forth in claim 29 in which one of said solitons
has a peak power which exceeds that of the other of said solitons during a substantial
part of the time that said solitons are propagating around said loop.
31. An optical fiber apparatus as set forth in claim 29 in which said lower order soliton
is an approximately first order soliton.
32. An optical fiber apparatus as set forth in claim 28 in which the dispersion of said
fiber decreases approximately continuously from said first end to said second end.
33. An optical fiber apparatus as set forth in claim 28 in which the dispersion of said
fiber decreases in a plurality of discrete steps from said first end to said second
end, each step comprising a fiber segment having a predetermined respective length
and an approximately constant respective dispersion.
34. An optical fiber apparatus as set forth in claim 33 in which the lengths of said segments
are small in relation to the local periods of said solitons.
35. An optical fiber apparatus as set forth in claim 28 in which the dispersion of said
fiber in ps/nm-km has a positive value in all parts of said loop.
36. An apparatus as set forth in claim 28 in which said pulse parameters further include
the direction of propagation of said counter propagating pulses.
37. An optical fiber apparatus as set forth in claim 28 in which said different order
solitons include a lower order component soliton and a higher order component soliton,
in which said loop parameters are such that said lower order component soliton returns
to said coupler with temporal width approximately equal to that with which it enter
said loop, in which said higher order soliton returns to said coupler with a temporal
width approximately equal to that of a lower order soliton, and in which said lower
and higher order solitons return to said coupler in generally out-of-phase relationship.
38. An optical fiber apparatus as set forth in claim 37 in which one of said solitons
has an amplitude which exceeds that of the other of said solitons for enough of its
propagation time to give rise to said generally out-of-phase relationship.
39. An optical fiber apparatus as set forth in claim 37 in which said lower order soliton
is an approximately first order soliton.
1. Optische Faservorrichtung zur Verwendung mit optischen Impulsen, welche aufweist:
a) eine optische Faser, welche ein erstes Ende enthält, welches eine relativ hohe
Dispersion hat, und ein zweites Ende enthält, welches eine relativ niedrige Dispersion
aufweist,
b) einen optischer Koppler, welcher erste und zweite E/A-Anschlüsse und erste und
zweite Ringanschlüsse hat, wobei die ersten und zweiten Ringanschlüsse mit den ersten
und zweiten Enden der Faser verbunden sind, um die Faser zu einem Ring zu formen,
welcher durch einen Satz von Ringparametern beschrieben wird, welche die Länge, die
effektive Fläche und die Dispersionswerte an dem ersten und zweiten Ende der Faser,
die den Ring aufweist, und die mathematische Form der monotonen Funktion aufweist,
welche die Dispersion der Faser beschreibt, wobei der Koppler als Vorrichtung dient
zum:
i) Aufteilen eines Eingangsimpulses, welcher durch einen Satz von Pulsparametern beschrieben
wird, welcher die Amplitude und die zeitliche Breite des Eingangspulses aufweist,
welcher in einen der E/A-Anschlüsse eintritt, in zwei Teilpulse, welche in Gegenrichtung
in dem Ring umlaufen und
ii) Empfängen von Teilpulsen, welche zu dem Koppler zurückkehren, nachdem sie in dem
Ring umgelaufen sind und Aufteilen der Energie der zurückkehrenden Teilpulse zwischen
den ersten und zweiten E/A-Anschlüssen entsprechend ihrer relativen Phasen.
c) dadurch gekennzeichnet, dass die optische Faser eine Dispersion hat, welche als eine monotone Funktion der Entfernung
von dem ersten Ende der Faser abnimmt;
d) wobei der Ring differentiell die gegeneinander laufenden Pulse so beeinflusst,
dass die Ringparameter die Energie der empfangenen Pulse dazu bringen, dass sie zwischen
den E/A-Anschlüssen entsprechend der Pulsparameter aufgeteilt werden.
2. Optische Faservorrichtung nach Anspruch 1, in welcher der optische Koppler ein Koppelverhältnis
von ungefähr 50:50 liefert.
3. Optische Faservorrichtung nach Anspruch 1, in welcher die Dispersion der Faser ungefähr
exponentiell vom ersten Ende bis zum zweiten Ende abnimmt.
4. Optische Faservorrichtung nach Anspruch 3, in welcher sich die Dispersion D der Faser
mit der Entfernung Z vom ersten Ende der Faser ungefähr entsprechend der Gleichung
ändert:
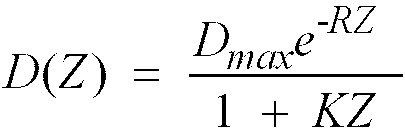
wobei D
MAX die Dispersion der Faser am ersten Ende ist, R das exponentielle Änderungsverhältnis
der Dispersion der Faser ist und K eine Konstante ist.
5. Optische Faservorrichtung nach Anspruch 1, in welcher die Dispersion der Faser in
einer Vielzahl von Schritten von dem ersten bis zum zweiten Ende abnimmt, wobei jeder
Schritt ein Fasersegment beinhaltet, welches eine vorher festgelegte, jeweilige Länge
und eine ungefähr konstante jeweilige Dispersion hat.
6. Optische Faservorrichtung nach Anspruch 1, in welcher die Dispersion der Faser ungefähr
kontinuierlich vom ersten bis zum zweiten Ende abnimmt.
7. Optische Faservorrichtung nach Anspruch 1, in welcher die Faser einen Kernteil, welcher
einen ersten Brechungsindex hat, und einen Durchmesser und ein Ummantelungsteil hat,
welches einen zweiten Brechungswinkel hat, und einen Außendurchmesser hat, welcher
ungefähr konstant ist, wobei der Durchmesser des Kernteils monoton vom ersten Ende
bis zum zweiten Ende abnimmt.
8. Optische Faservorrichtung nach Anspruch 7, in welcher die Unterschiede zwischen dem
ersten und dem zweiten Brechungsindex aus dem Unterschied zwischen den Konzentrationen
der Dotiermittel in dem Kernteil und dem Mantelteil herrühren, und wobei sich der
Pegel bzw. Grad an Dotiermittel von wenigstens einem der Teile monoton vom ersten
bis zum zweiten Ende ändert.
9. Optische Faservorrichtung nach Anspruch 1, in welcher die Dispersionen am ersten und
zweiten Ende der Faser derartige Werte haben, so dass Eingangspulse, welche gebräuchliche
Kombinationen von Spitzenleistung und zeitlicher Breite haben, in diesem Ring als
Solitone umlaufen.
10. Optische Faservorrichtung nach Anspruch 5, in welcher die Dispersion am ersten und
zweiten Ende der Faser derartige Werte hat, so dass Eingangspulse, welche gebräuchliche
Kombinationen von Spitzenleistung und zeitlicher Breite aufweisen in dem Ring als
Solitone umlaufen.
11. Optische Faservorrichtung nach Anspruch 10, in welcher die Länge der Segmente klein
im Verhältnis zu den lokalen Perioden der Solitone sind.
12. Optische Faservorrichtung nach Anspruch 10, in welcher die Anzahl der Segmente groß
in bezug auf die lokalen Perioden der Solitone sind.
13. Optische Faservorrichtung nach Anspruch 1, in welcher die Faser eine Dispersion in
dem anormalen Dispersionssystem hat.
14. Optische Faservorrichtung nach Anspruch 1, wobei wenigstens die Dispersionswerte am
ersten und zweiten Ende der Faser, die den Ring aufweist, und die mathematische Form
der monotonen Funktion, welche die Dispersion der Faser beschreibt, so gewählt werden,
so dass ein Ring geliefert wird, in welchem
ein Puls, welcher eine zeitliche Breite größer als eine vorher ausgewählte Breite
hat, beim Eintreten in den Ring eine Phase hat, welche im wesentlichen die gleiche
Pulsphase nach dem Lauf durch den Ring ist, und
ein Puls, welcher eine zeitliche Breite kleiner als die vorher ausgewählte Breite
hat, eine Phase beim Eintreten in den Ring hat, welche unterschiedlich von der Pulsphase
nach dem Lauf durch den Ring ist.
15. Optische Faservorrichtung nach Anspruch 14, in welcher die Breiten der Pulse, welche
zeitliche Breiten größer als die vorher festgelegten zeitlichen Breiten haben, groß
im Vergleich zur Länge des Ringes sind.
16. Optische Faservorrichtung nach Anspruch 14, in welcher die Eingangspulse, welche zeitliche
Breiten kleiner als die vorher festgelegte zeitliche Breite haben, eine Reaktionslänge
haben, welche verhältnismäßig kurz im Vergleich zur Länge des Ringes ist, und in welcher
Eingangspulse, welche zeitliche Breiten größer als die vorher festgelegte zeitliche
Breite haben, eine Reaktionslänge haben, welche verhältnismäßig lang im Verhältnis
zur Länge des Ringes ist.
17. Optische Faservorrichtung nach Anspruch 14, in welcher der Koppler einen Eingangspuls,
welcher eine zeitliche Breite kleiner als die vorher festgelegte zeitliche Breite
hat, in ein Einzelsoliton mit niedrigerer Ordnung und ein Einzelsoliton mit höherer
Ordnung aufteilt, wobei das Einzelsoliton mit höherer Ordnung, eine Amplitude aufweist,
welche das Einzelsoliton der niedrigeren Ordnung während eines wesentlichen Teils
an Zeit, bei welcher die Solitone in den Ring umlaufen, übersteigt.
18. Optische Faservorrichtung nach Anspruch 17, in welcher der Koppler einen Eingangspuls,
welcher eine zeitliche Breite größer als die vorher festgelegte zeitliche Breite hat,
in Solitone mit der gleichen Ordnung aufteilt.
19. Optische Faservorrichtung nach Anspruch 17, in welcher die Dispersion der Faser ungefähr
kontinuierlich von dem ersten bis zum zweiten Ende abnimmt.
20. Optische Faservorrichtung nach Anspruch 17, in welcher die Dispersion der Faser sich
in einer Vielzahl von Schritten ändert, wobei jeder Schritt ein Fasersegment aufweist,
welches eine vorher festgelegte jeweilige Länge und eine ungefähr konstante jeweilige
Dispersion aufweist.
21. Optische Faservorrichtung nach Anspruch 20, in welcher die Längen der Segmente klein
im Verhältnis zu den lokalen Perioden der Solitone sind.
22. Optische Faservorrichtung nach Anspruch 20, in welcher die Eingangspulse, welche zeitliche
Breiten größer als die vorher festgelegte zeitliche Breite haben, im wesentlichen
auf die durchschnittlichen Dispersionswerte der Stufen reagieren, und die Eingangspulse,
welche zeitliche Breiten kleiner als die vorher festgelegte zeitliche Breite haben,
auf die individuellen Dispersionswerte der Stufen reagieren.
23. Optische Faservorrichtung nach Anspruch 20, in welcher die Dispersionswerte der Schritte
exponentiell vom ersten bis zum zweiten Ende abnehmen.
24. Optische Faservorrichtung nach Anspruch 14, in welcher die Faser eine Dispersion im
anomalen Dispersionssystem aufweist.
25. Optische Faservorrichtung nach Anspruch 14, wobei die Teilpulse, welche eine zeitliche
Breite kleiner als die vorher ausgewählte Breite haben, Solitone der gleichen oder
unterschiedlicher Ordnung sind.
26. Optische Faservorrichtung nach Anspruch 25, in welcher die Solitone ein Soliton niedrigerer
Ordnung und ein Soliton höherer Ordnung beinhalten und wobei das Soliton höherer Ordnung
eine Amplitude aufweist, welche die des Solitons niedrigerer Ordnung während einem
wesentlichen Teil an Zeit, während der die Solitone in dem Ring umlaufen, übersteigt.
27. Optische Faservorrichtung nach Anspruch 25, in welcher die Dispersionswerte der Schritte
exponentiell von dem ersten Ende bis zu dem zweiten Ende abnehmen.
28. Optische Faservorrichtung nach Anspruch 1, wobei die Ringparameter ferner die Dämpfung
und die effektive Fläche der Faser und die Beziehung zwischen den Parametern, welche
die Dämpfung, die effektive Fläche, die Dispersion der Faserenden beinhalten, und
die Abhängigkeit der Dispersion von der Entfernung vom ersten Faserende beinhalten,
derart, dass,
Pulse, welche eine Amplitude unterhalb einer vorausgewählten Amplitude haben, in zwei
Teilpulse aufgeteilt werden, welche nicht Solitone sind und welche eine Phase haben,
welche im wesentlichen die gleiche vor und nach dem Umlauf durch den Ring bleibt und
Pulse, welche eine Amplitude oberhalb der vorausgewählten Amplitude haben, in Teilpulse
aufgeteilt werden, welche Solitone von verschiedener oder der gleichen Ordnung sind
und welche eine Phase haben, welche sich beim Umlauf durch den Ring ändert.
29. Optische Faservorrichtung nach Anspruch 28, in welcher die Solitone unterschiedlicher
Ordnung ein Einzelsoliton niedrigerer Ordnung beinhalten und ein Einzelsoliton höherer
Ordnung, wobei das Einzelsolitone niedrigerer Ordnung zum Koppler mit einer zeitlichen
Breite zurückkehrt, welche ungefähr gleich der ist, mit welcher es in den Kreis eintrat,
wobei das Ein-Zelsoliton höherer Ordnung zum Koppler mit einer Breite zurückkehrt,
welche ungefähr gleich der des Einzelsolitons mit niedrigerer Ordnung ist und wobei
das Soliton niedrigerer und höherer Ordnung zum Koppler in einer im allgemeinen nicht
in Phase Beziehung zurückkehrt.
30. Optische Faservorrichtung nach Anspruch 29, wobei eines der Solitone eine Spitzenleistung
hat, welche die des anderen der Solitone innerhalb eines wesentlichen Teils an Zeit,
währenddem die Solitone innerhalb des Ringes umlaufen, übersteigt.
31. Optische Faservorrichtung nach Anspruch 29, wobei das Soliton niedrigerer Ordnung
ein Soliton nahezu erster Ordnung ist.
32. Optische Faservorrichtung nach Anspruch 28, wobei die Dispersion der Faser ungefähr
kontinuierlich vom ersten Ende bis zum zweiten Ende abnimmt.
33. Optische Faservorrichtung nach Anspruch 28, wobei die Dispersion der Faser in einer
Vielzahl von diskreten Schritten vom ersten Ende bis zum zweiten Ende abnimmt, wobei
jeder Schritt ein Fasersegment aufweist, welches eine vorher festgelegte jeweilige
Länge und eine ungefähr konstante jeweilige Dispersion aufweist.
34. Optische Faservorrichtung nach Anspruch 33, wobei die Längen der Segmente klein im
Verhältnis zu den lokalen Perioden der Solitone sind.
35. Optische Faservorrichtung nach Anspruch 28, wobei die Dispersion der Faser in ps/nn-km
einen positiven Wert in allen Teilen des Ringes hat.
36. Optische Faservorrichtung nach Anspruch 28, wobei die Pulsparameter ferner die Ausbreitungsrichtung
der entgegengesetzt umlaufenden Pulse einschliessen.
37. Optische Faservorrichtung nach Anspruch 28, wobei die Solitone unterschiedlicher Ordnung
ein Einzelsoliton niedrigerer Ordnung und ein Einzelsoliton höherer Ordnung beinhalten,
wobei die Ringparameter derart sind, dass das Einzelsoliton niedrigerer Ordnung zu
dem Koppler mit einer zeitlichen Breite zurückkehrt, welche ungefähr gleich derjenigen
ist, mi welcher es in den Ring eintritt, wobei das Soliton höherer Ordnung zu dem
Koppler mit einer zeitlichen Breite zurückkehrt, welche ungefähr gleich dem Soliton
niedrigerer Ordnung ist und wobei die Solitone niedrigerer und höherer Ordnung zu
dem Koppler im allgemeinen in einer nicht in Phase Beziehung zurückkehren.
38. Optische Faservorrichtung nach Anspruch 37, wobei eines der Solitone eine Amplitude
hat, welche die des anderen der Solitone um ausreichend seiner Ausbreitungszeit bzw.
Laufzeit übersteigt, um allgemein diese nicht in Phase Beziehung hervorzurufen.
39. Optische Faservorrichtung nach Anspruch 37, wobei das Soliton niedrigerer Ordnung
nahezu ein Soliton erster Ordnung ist.
1. Un appareil à fibre optique destiné à être utilisé avec des impulsions optiques, comprenant
:
a) une fibre optique présentant une première extrémité offrant une dispersion relativement
forte et une deuxième extrémité offrant une dispersion relativement faible,
b) un coupleur optique comportant un premier et un deuxième ports d'entré/sortie et
un premier et un deuxième ports de boucle, lesdits premier et deuxième ports de boucle
étant reliés aux première et deuxième extrémités de ladite fibre pour faire former
à ladite fibre une boucle qui est définie par un ensemble de paramètres de boucle
comprenant la longueur, la section efficace et les valeurs de dispersion aux première
et deuxième extrémités de ladite fibre comprenant ladite boucle, et la forme mathématique
de ladite fonction monotone, qui définit la dispersion de ladite fibre, ledit coupleur
servant de moyen pour :
i) diviser une impulsion d'entrée qui est définie par un ensemble de paramètres d'impulsion
comprenant l'amplitude et la largeur temporelle de ladite impulsion d'entrée admise
à l'un desdits ports d'entré/sortie en des impulsions composantes qui se propagent
en sens contraires sur ladite boucle, et
ii) recevoir les impulsions composantes revenant audit coupleur après circulation
sur ladite boucle et répartition de l'énergie des impulsions composantes en retour
entre lesdits premier et deuxième ports d'entré/sortie en fonction de leur phase relative
;
c) caractérisé en ce que la fibre optique a une dispersion qui décroît selon une fonction monotone de la distance
à ladite première extrémité de ladite fibre ;
d) ladite boucle affectant différentiellement lesdites impulsions se propageant en
sens contraires de sorte que lesdits paramètres de boucle entraînent la répartition
de l'énergie des impulsions reçues entre lesdits ports d'entré/sortie en conformité
avec lesdits paramètres d'impulsion.
2. Un appareil à fibre optique selon la revendication 1 dans lequel ledit coupleur optique
fournit un rapport de couplage d'environ 50:50.
3. Un appareil à fibre optique selon la revendication 1 dans lequel la dispersion de
ladite fibre décroît selon une loi approximativement exponentielle de ladite première
extrémité à ladite deuxième extrémité.
4. Un appareil à fibre optique selon la revendication 3 dans lequel la dispersion D de
ladite fibre varie avec la distance Z comptée depuis la première extrémité de ladite
fibre en conformité approximative avec l'équation ;
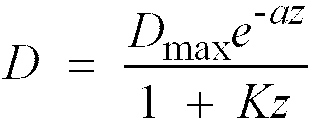
où D
MAX est la dispersion de ladite fibre optique à ladite première extrémité, R est l'allure
de variation exponentielle de la dispersion de ladite fibre et K est une constante.
5. Un appareil à fibre optique selon la revendication 1 dans lequel la dispersion de
ladite fibre décroît en une pluralité de paliers de ladite première extrémité à ladite
deuxième extrémité, chaque palier comprenant un segment de fibre présentant une longueur
respective prédéterminée et une dispersion respective approximativement constante.
6. Un appareil à fibre optique selon la revendication 1 dans lequel la dispersion de
ladite fibre décroît de manière approximativement continue de ladite première extrémité
à ladite deuxième extrémité.
7. Un appareil à fibre optique selon la revendication 1 dans lequel ladite fibre comporte
une portion de coeur présentant un premier indice de réfraction et un diamètre et
une portion de gainage présentant un deuxième indice de réfraction, et un diamètre
extérieur qui est approximativement constant, le diamètre de ladite portion de coeur
décroissant de façon monotone de ladite première extrémité à ladite deuxième extrémité.
8. Un appareil à fibre optique selon la revendication 7 dans lequel les différences entre
lesdits premier et deuxième indice de réfraction résultent de différences entre les
concentrations en agents dopants dans ladite portion de coeur et dans ladite portion
de gainage, et dans lequel la teneur en agent dopant de l'une au moins desdites portions
varie de façon monotone de ladite première extrémité à ladite deuxième extrémité.
9. Un appareil à fibre optique selon la revendication 1 dans lequel les dispersions aux
première et deuxième extrémités de ladite fibre ont des grandeurs telles que des impulsions
d'entrée offrant des combinaisons appropriées de puissance de crête et de largeur
temporelle se propagent dans ladite boucle sous forme de solitons.
10. Un appareil à fibre optique selon la revendication 5 dans lequel les dispersions aux
première et deuxième extrémités de ladite fibre ont des grandeurs telles que les impulsions
d'entrée offrant des combinaisons appropriées de puissance de crête et de largeur
temporelle se propagent dans ladite boucle sous forme de solitons.
11. Un appareil à fibre optique selon la revendication 10 dans lequel les longueurs desdits
segments sont petites par rapport aux périodes locales desdits solitons.
12. Un appareil à fibre optique selon la revendication 10 dans lequel le nombre desdits
segments est grand par rapport aux périodes locales desdits solitons.
13. Un appareil à fibre optique selon la revendication 1 dans lequel ladite fibre présente
une dispersion se situant dans le domaine des dispersions anormales.
14. Un appareil à fibre optique selon la revendication 1 dans lequel au moins les valeurs
de dispersion aux première et deuxième extrémités de ladite fibre comprenant ladite
boucle, et la forme mathématique de ladite fonction monotone, qui décrit la dispersion
de ladite fibre sont choisies de façon à fournir une boucle dans laquelle
une impulsion, ayant une largeur temporelle supérieure à une largeur présélectionnée,
a une phase à son entrée dans la boucle sensiblement identique à la phase de l'impulsion
après propagation à travers la boucle, et
une impulsion, ayant une largeur temporelle inférieure à ladite largeur présélectionnée,
a une phase à son entrée dans la boucle différente de la phase de l'impulsion après
propagation à travers la boucle.
15. Un appareil à fibre optique selon la revendication 14 dans lequel les largeurs des
impulsions ayant des largeurs temporelles supérieures à ladite largeur temporelle
prédéterminée sont grandes par rapport à la longueur de ladite boucle.
16. Un appareil à fibre optique selon la revendication 14 dans lequel les impulsions d'entrée
ayant des largeurs temporelles inférieures à ladite largeur temporelle prédéterminée
ont une longueur de réaction qui est relativement courte par rapport à la longueur
de ladite boucle, et dans lequel les impulsions d'entrée ayant des largeurs temporelles
supérieures à ladite largeur temporelle prédéterminée ont une longueur de réaction
qui est relativement grande par rapport à la longueur de ladite boucle.
17. Un appareil à fibre optique selon la revendication 14 dans lequel ledit coupleur divise
une impulsion d'entrée ayant une largeur temporelle inférieure à ladite largeur temporelle
prédéterminée en un soliton composant d'ordre inférieur et en un soliton composant
d'ordre supérieur, ledit soliton composant d'ordre supérieur ayant une amplitude qui
dépasse celle dudit soliton composant d'ordre inférieur pendant une partie substantielle
du temps pendant lequel lesdits solitons circulent sur ladite boucle.
18. Un appareil à fibre optique selon la revendication 17 dans lequel ledit coupleur divise
une impulsion d'entrée ayant une largeur temporelle supérieure à ladite largeur temporelle
prédéterminée en des solitons du même ordre.
19. Un appareil à fibre optique selon la revendication 17 dans lequel la dispersion de
ladite fibre décroît de manière approximativement continue de ladite première extrémité
à ladite deuxième extrémité.
20. Un appareil à fibre optique selon la revendication 17 dans lequel la dispersion de
ladite fibre varie en une pluralité de paliers, chaque palier comprenant un segment
de fibre présentant une longueur respective prédéterminée et une dispersion respective
approximativement constante.
21. Un appareil à fibre optique selon la revendication 20 dans lequel les longueurs desdits
segments sont petites par rapport aux périodes locales desdits solitons.
22. Un appareil à fibre optique selon la revendication 20 dans lequel des impulsions d'entrée
ayant des largeurs temporelles supérieures à ladite largeur temporelle prédéterminée
répondent substantiellement à la moyenne des valeurs de dispersion desdits paliers,
et des impulsions d'entrée ayant des largeurs temporelles inférieures à ladite largeur
temporelle prédéterminée répondent aux valeurs de dispersion individuelles desdits
paliers.
23. Un appareil à fibre optique selon la revendication 20 dans lequel les valeurs de dispersion
desdits paliers décroissent exponentiellement de ladite première extrémité à ladite
deuxième extrémité.
24. Un appareil à fibre optique selon la revendication 14 dans lequel ladite fibre présente
une dispersion se situant dans le domaine des dispersions anormales.
25. Un appareil à fibre optique selon la revendication 14 dans lequel lesdites impulsions
composantes, ayant une largeur temporelle inférieure à ladite largeur présélectionnée,
sont des solitons d'ordre identique ou différent.
26. Un appareil à fibre optique selon la revendication 25 dans lequel lesdits solitons
comprennent un soliton d'ordre inférieur et un soliton d'ordre supérieur et dans lequel
ledit soliton d'ordre supérieur a une amplitude qui dépasse celle dudit soliton d'ordre
inférieur pendant une partie substantielle du temps pendant lequel lesdits solitons
circulent sur ladite boucle.
27. Un appareil à fibre optique selon la revendication 25 dans lequel les valeurs de dispersion
desdits paliers décroissent exponentiellement de ladite première extrémité à ladite
deuxième extrémité.
28. Un appareil à fibre optique selon la revendication 1 dans lequel lesdits paramètres
de boucle comprennent en outre l'atténuation et la section efficace de ladite fibre
et la relation entre lesdits paramètres comprenant l'atténuation, la section efficace,
la dispersion auxdites extrémités de la fibre et la dépendance de la dispersion vis-à-vis
de la distance à ladite première extrémité de fibre, est telle que,
les impulsions ayant une amplitude inférieure à une amplitude présélectionnée se
trouvent divisées en des impulsions composantes qui ne sont pas des solitons et qui
ont une phase qui demeure sensiblement la même avant et après circulation sur ladite
boucle, et
les impulsions ayant une amplitude supérieure à l'amplitude présélectionnée se
trouvent divisées en des impulsions composantes qui sont des solitons d'ordre identique
ou différent et qui ont une phase qui change au cours de la circulation sur ladite
boucle.
29. Un appareil à fibre optique selon la revendication 28 dans lequel lesdits solitons
d'ordres différents comprennent un soliton composant d'ordre inférieur et un soliton
composant d'ordre supérieur, dans lequel ledit soliton composant d'ordre inférieur
retourne audit coupleur avec une largeur temporelle approximativement égale à celle
avec laquelle il a été admis dans ladite boucle, dans lequel ledit soliton composant
d'ordre supérieur retourne audit coupleur avec une largeur temporelle approximativement
égale à celle dudit soliton d'ordre inférieur et dans lequel les solitons d'ordre
inférieur et d'ordre supérieur retournent audit coupleur globalement en discordance
de phase.
30. Un appareil à fibre optique selon la revendication 29 dans lequel l'un desdits solitons
présente une puissance de crête qui dépasse celle de l'autre soliton durant une partie
substantielle du temps pendant lequel lesdits solitons circulent sur ladite boucle.
31. Un appareil à fibre optique selon la revendication 29 dans lequel ledit soliton d'ordre
inférieur est un soliton approximativement du premier ordre.
32. Un appareil à fibre optique selon la revendication 28 dans lequel la dispersion de
ladite fibre décroît de manière approximativement continue de ladite première extrémité
à ladite deuxième extrémité.
33. Un appareil à fibre optique selon la revendication 28 dans lequel la dispersion de
ladite fibre diminue en une pluralité de paliers discrets de ladite première extrémité
à ladite deuxième extrémité, chaque palier comprenant un segment de fibre présentant
une longueur respective prédéterminée et une dispersion respective approximativement
constante.
34. Un appareil à fibre optique selon la revendication 33 dans lequel les longueurs desdits
segments sont petites par rapport aux périodes locales desdits solitons.
35. Un appareil à fibre optique selon la revendication 28 dans lequel la dispersion de
ladite fibre en ps/nm/km a une valeur positive dans toutes les parties de ladite boucle.
36. Un appareil à fibre optique selon la revendication 28 dans lequel lesdits paramètres
d'impulsion comprennent en outre le sens de propagation desdites impulsions se propageant
en sens contraires.
37. Un appareil à fibre optique selon la revendication 28 dans lequel lesdits solitons
d'ordres différents comprennent un soliton composant d'ordre inférieur et un soliton
composant d'ordre supérieur, dans lequel lesdits paramètres de boucle sont tels que
ledit soliton composant d'ordre inférieur retourne audit coupleur avec une largeur
temporelle approximativement égale à celle avec laquelle il a été admis dans ladite
boucle, dans lequel ledit soliton d'ordre supérieur retourne audit coupleur avec une
largeur temporelle approximativement égale à celle d'un soliton d'ordre inférieur,
et dans lequel lesdits solitons d'ordre inférieur et d'ordre supérieur retournent
audit coupleur globalement en discordance de phase.
38. Un appareil à fibre optique selon la revendication 37 dans lequel l'un desdits solitons
a une amplitude qui dépasse celle de l'autre desdits solitons pendant une fraction
suffisante de son temps de propagation pour donner naissance à ladite discordance
de phase globale.
39. Un appareil à fibre optique selon la revendication 37 dans lequel ledit soliton d'ordre
inférieur est un soliton approximativement du premier ordre.