TECHNICAL FIELD OF THE INVENTION
[0001] The present invention relates to a permanent magnet alloy based on R (wherein R represents
yttrium (Y) or a rare-earth element), boron (B), carbon (C), cobalt (Co) and iron
(Fe), which exhibits particularly improved heat resistance as such that little degradation
occurs on the magnetic force even in case it is used under ambient at a temperature
as high as 200 °C.
BACKGROUND OF THE INVENTION
[0002] A Sm-Co based magnet is known as a rare-earth magnet having improved heat resistance,
but is expensive. The term "heat resistance" as referred herein particularly signifies
that the magnetic force of the magnet does not degrade by heat. As disclosed in Japanese
Patent Public Disclosure No. 4-116144 (Patent No. 2740981), one of inventors of the
present invention and others have proposed a R-B-C-Co-Fe based permanent magnet alloy
as a rare-earth magnet reduced in cost and yet improved in heat resistance. This magnet
alloy contains carbon (C) as an essential alloy element, and utilizes a combination
of a light rare-earth element and a heavy rare-earth element for the rare-earth element
(R). The disclosure teaches that the irreversible demagnetization of the magnet alloy
is improved remarkably (i.e., the negative irreverible demagnetization values approach
0%) by the incorporation of C, and that the irreversible demagnetization is further
improved by partly incorporating a heavy rare-earth element for R.
OBJECT OF THE INVENTION
[0003] In case a permanent magnet is assembled in appliances that are installed in the vicinity
of a heat-emitting source, it is essential that the magnetic force of the permanent
magnet does not drop when brought to higher temperatures, i.e., that the residual
magnetic flux density (Br) does not undergo degradation when heated. However, there
are cases in which the magnet is used under conditions as such that the operation
temperature approaches ca. 200 °C (e.g., automobile engine appliances are operated
at ca. 200 °C, and as a matter of course, the same holds true for motors of electric
automotive vehicles). Then, Sm-Co based magnets are the only type of known magnets
applicable to this field. However, as stated above, Sm-Co based magnets are expensive,
and the ordinary Nd-Fe(Co)-B based rare-earth magnets are unfeasible for such high
temperature (e.g., 200 °C) applications.
[0004] Although the aforementioned Japanese Patent Public Disclosure No. 4-116144 teaches
that the incorporation of C (carbon) as the alloying element in a permanent magnet
improves the irreversible demagnetization and that the partial replacement of R by
a heavy rare-earth element further improves the irreversible demagnetization, there
is not shown any magnet that does not undergo demagnetization when heated to 200 °C.
[0005] In the light of such circumstances, an object of the present invention is to provide
a permanent magnet having improved heat resistance feasible for use at such a high
temperature of 200 °C, yet at a low production cost.
SUMMARY OF THE INVENTION
[0006] In order to achieve the object above based on the fundamental concept of incorporating
C to improve the heat resistance of the permanent magnet alloy, as proposed in Japanese
Patent Public Disclosure No. 4-116144, the present inventors investigated and studied
the influence of each of the heavy rare-earth elements on the heat resistance. As
a result, in addition to the incorporation of the basic rare-earth elements such as
Nd and Pr, it has been newly found that the addition of Dy and Tb in combination and
in proper quantities, particularly when they are added in relation to each other,
greatly improves the heat resistance of the permanent magnet.
[0007] Thus, in accordance with the present invention, there is provided a permanent magnet
alloy having an improved heat resistance as set forth in claim 1.
[0008] Also provided is a process for producing such a permanent magnet alloy as set forth
in claim 7.
[0009] Preferred embodiments of the present invention may be gathered from the dependent
claims.
BRIEF EXPLANATION OF THE DRAWINGS
[0010]
FIG. 1 is a diagram showing the distribution of irreversible demagnetization, in which
the values of irreversible demagnetization at 200 °C of the magnets shown in Table
2 are arranged in terms of the content of Dy and Tb;
FIG. 2 is a diagram showing the observed irreversible demagnetization values at differing
temperatures for the magnet described in Example 24 disclosed in Japanese Patent Public
Disclosure No. 4-116144 and those of Example 2 according to the present invention,
in which the specimens are each shaped as such that the permeance coefficient (Pc)
be 3 and magnetized by applying 50 KOe; and
FIG. 3 is a diagram showing the observed irreversible demagnetization values similar
to those shown in Fig. 2, except that the specimens are shaped as such that they may
yield a permeance coefficient (Pc) of 1.
DESCRIPTION OF THE PREFERRED EMBODIMENTS
[0011] In designing appliances which may be operated under cases as such that the magnet
assembled therein is brought at a temperature of 200 °C, the irreversible demagnetization
at 200 °C serves as an index. More specifically, it is preferred to select a design
that the value (a negative value) of irreversible demagnetization (200 °C) according
to equation
(1) above approaches 0 % as possible.
[0012] When a proper amount of C is incorporated into a R-B-Co-Fe based sintered alloy,
where R is representatively Nd or a combination of Nd and Pr, the value (negative
value) of irreversible demagnetization (160 °C) approaches zero. This fact is shown
in the example of Japanese Patent Public Disclosure No. 4-116144. However, the irreversible
demagnetization (160 °C) described in this disclosure is obtained by replacing the
value of A
200 by A
160 (where A
160 is the flux value of a magnet measured on a specimen maintained at 160 °C for a duration
of 120 minutes and then cooled to room temperature), and is an observed value for
a permeance coefficient (Pc) of 3. That is, the irreversible demagnetization (160
°C) is a value obtained by magnetizing a specimen shaped as such that Pc be 3 and
magnetized at 50 KOe, and by measuring the flux values A
25 and A
160. As described in the disclosure, the incorporation of C is known to be effective
for improving the heat resistance (and for imparting resistance against oxidation).
However, nothing is known about the irreversible demagnetization at 200 °C. Furthermore,
in the existing R-(Fe,Co)-B based sintered magnet alloys (which do not contain C as
the alloy element), none is found to yield an irreversible demagnetization (200 °C)
in a range of 0% to -20 %.
[0013] Since the proposal of the disclosure above, the present inventors have continued
various types of tests and researches on the composition of the alloy and the process
for the production thereof with an aim to improve the heat resistance of the R-Fe-Co-C-B
based sintered magnet alloys, and have found that the combined addition of Dy and
Tb in proper quantities results in a magnet alloy having a considerably low irreversible
demagnetization. The effect of the addition is not remarkable when Dy or Tb is added
independent to each other, but a favorable heat resistance is achieved in case the
both are added in combination.
[0014] The reason for confining the content of each of the components constituting the magnet
allow according to the present invention is outlined below, together with the process
for producing the alloy magnet according to the present invention. In the following
description, " at.% signifies % by atomic, that is percentage on an atomic basis".
C: 0.1 - 15 at.%
[0015] As described in Japanese Patent Public Disclosure No. 4-116144, the disadvantageous
characteristic of a rare-earth magnet. i.e., the tendency prone to oxidation, can
be modified by the addition of C while favorably maintaining the magnetic properties
of the magnet alloy. Carbon also contributes to the reduction of irreversible demagnetization.
If the addition of C is less than 0.1 at.%, the effect thereof on improving the oxidation-resistance
and the heat resistance is not sufficient. If the addition of C should exceed 15 at.%,
on the other hand, the value of Br begins to drop. Accordingly, C is incorporated
at a content in a range of 0.1 to 15 at.%, but a preferred range is 1.0 - 10 at.%,
and more preferably, is in the range of 2.5 to 7 at.%.
B: 0.5 to 15 at.%
[0016] Boron (B) is necessary for the formation of a magnetic phase, and it should be present
at least 0.5 at.%. However, the addition of B in excessive amount reversibly deteriorates
the magnetic properties. Accordingly, B is added in an amount of 0.5 to 15 at.%, preferably
1.0 to 10 at.%, and more preferably, 1.5 to 7 at.%.
C and B in total: 2 to 30 at.%
[0017] For the formation of a magnetic phase and the improvement of the resistance against
oxidation, C and B in total must account for at least 2 at.%. However, the incorporation
of C and B in total exceeding 30 at.% impairs the magnetic properties; accordingly,
C and B in total must account for 2 to 30 at.%.
Co: 40 at.% or less
[0018] Cobalt elevates the Curie point while maintaining the magnetic properties. Thus,
the addition of Co is essential, but an addition thereof in an amount exceeding 40
at.% considerably decreases the coercive force of the magnet. Accordingly, the addition
of Co must be 40 at.% or less.
Dy and Tb in total: 0.5 to 5 at.%
[0019] Dysprosium (Dy) and terbium (Tb) are the characteristic elements of the magnet of
the present invention, and their combined addition remarkably lowers the irreversible
demagnetization. For this purpose, Dy and Tb must be added at an amount of 0.5 at.%
or more in total, but their effect on improving the heat resistance saturates if a
total addition of them is in excess of 5 at.% and it may reversibly affect the magnetic
properties. Accordingly, their addition in total must be 0.5 to 5 at. %. As shown
hereinafter in the comparative example, the addition of Dy or Tb alone does not contribute
to the reduction of irreversible demagnetization. Presumably, Dy and Tb function synergetically
to lower the irreversible demagnetization. Furthermore, those elements are preferably
added at a Tb(at.%)/Dy( at.%) ratio, expressed by percentage on atomic basis, in the
range of 0.1 to 0.8. As shown in the examples hereinafter, the addition of 0.3 to
4.9 at.% of Dy and 0.1 to 4.7 at.% of Tb enables a magnet having improved heat resistance
with a irreversible demagnetization at 200 °C at a permeance coefficient of 1 in a
range of 0 to -20%, preferably 0 to -15%.
R: 8 to 20 at.%
[0020] As rare-earth elements other than Dy and Tb, at least one element selected from the
group consisting of Nd, Pr, Ce, La, Y, Gd, Ho, Er and Tm may be added either singly
or in combination thereof at an amount of 8 to 20 at.%. By the addition of R, a magnetic
phase and a grain boundary phase are developed in the sintered magnet alloy to maintain
iHc and Br at high values. Among the elements for R, particularly preferred are Nd
and Pr, and the addition of Nd alone or a combination of Nd with Pr is most preferred.
If the addition of R is less than 8 at.%, a sufficient high Br cannot be achieved,
and the addition of R in excess of 20 at.% results in an insufficient value of Br.
A preferred range of R is 13 to 18 at.%.
[0021] A permanent magnet alloy according to the present invention having the composition
above yields an irreversible demagnetization (200 °C) in accordance with equation
(1) above at a low level as such in a range of 0 to -20 %, preferably 0 to -15 %,
and most preferably, 0 to -5 %. Thus, the present invention provides for the first
time, a permanent magnet alloy other than a Sm-Co based magnet suitable for high temperature
use. For high temperature applications, known boron-containing rare-earth magnets
having higher coercive force were used by taking the demagnetization at higher temperatures
into account. However, since the magnet according to the present invention is almost
free of demagnetization even at elevated temperatures, it can be used as it is as
a permanent magnet having a high magnetic force. In particular, the magnet according
to the present invention can maintain the magnetic properties for use at elevated
temperatures at an iHc of 13 KOe or higher, and preferably 15 KOe or higher. Considering
that the existing magnets required a considerably high iHc for use in applications
at elevated temperatures, the magnet according to the present invention can be regarded
as an effective permanent magnet alloy.
[0022] The permanent magnet alloy according to the present invention can be produced by
a process consisting of sequential steps of melting, casting, crushing, molding, and
sintering. As a melt casting process, there can be employed processes such as vacuum
melting and casting, melting and casting under an inert gas atmosphere, quench rolling,
atomization, etc. In order to obtain a sintered magnet having improved magnetic characteristics
and heat resistance, it is preferred to incorporate a step of heat treatment between
the steps of casting and crushing so as to subject the product before crushing to
a heat treatment at a temperature of 600 °C or higher under an inert gas atmosphere.
In this manner, the irreversible demagnetization can be further lowered. In the sintering
step, it is preferred to sinter the molding in the temperature range of 1,000 to 1,200
°C under an inert gas atmosphere and gradually cooling from the sintering temperature
to a temperature in a range of 600 to 900 °C, followed by quenching therefrom. The
irreversible demagnetization can be further lowered by the quenching performed after
the sintering.
[0023] The sintered magnet alloy according to the present invention can be produced in accordance
with the production method for a sintered magnet described in Japanese Patent Public
Disclosure No. 4-116144, except for the heat treatment and the quench treatment after
the sintering described above. The process of production is outlined below.
[0024] The raw materials weighed as such to yield the desired alloy composition are molten
in a vacuum melting furnace at a temperature of 1,600 °C or higher, and are casted
by quenching in a water-cooled casting mold. As described above, the cast ingot thus
obtained is thermally treated under gaseous Ar at a temperature of 600 °C or higher,
and subjected to coarse crushing by using a jaw crusher. The coarse-grained powder
thus prepared was finely milled by using a vibration ball mill to obtain a powder
consisting of particles having an average diameter in a range of 2 to 10 µm. Those
steps for such size reduction are carried out under gaseous Ar atmosphere. A part
of the raw material for C can be added in the latter step of milling. That is, a part
of the raw material for C is charged into the vacuum melting furnace, and the rest
is added in this step of fine milling. Carbon black is suitable for use as the raw
material of C, but also usable are organic materials containing C, such as an aliphatic
hydrocarbon, a higher fatty acid alcohol, a higher fatty acid, a higher fatty acid
amide, a metal soap, a fatty acid ester, etc.
[0025] Then, the powder thus obtained is compaction molded while applying an external magnetic
field. The preferred range for the molding pressure is 1 to 5 t/cm
2. and that for the external magnetic field is 15 KOe or higher. The molding step also
is preferably carried out under a gaseous Ar atmosphere. The molded product thus obtained
is then sintered under gaseous Ar in the temperature range of 1,000 to 1,200 °C for
a duration of about 2 hours. Then, as stated above, the resulting product is gradually
cooled to a temperature in the range of 600 to 900 °C, and quenched from the temperature.
To initiate the quenching from a temperature in the range of 600 to 900 °C, there
can be employed a method of spraying a low temperature inert gas or a method of immersing
the sintered product into water, an oil, or a liquid similar thereto, and preferably,
rapid cooling is performed from the quench initiation temperature in the range of
600 to 900 °C to a temperature of 400 °C or lower at a cooling rate of -50 °C/min
or higher, preferably -100 °C/min or higher.
[0026] Thus, in accordance with the present invention, there is provided a process for producing
a permanent magnet alloy having an improved heat resistance comprising melting and
casting each of the raw materials of the alloying elements, subjecting the resulting
alloy to pulverizing, compaction molding the resulting powder, and sintering the molding
under an inert gas atmosphere in a temperature range of 1,000 to 1,200 °C to obtain
a sintered magnet alloy, characterized in that the alloy before pulverizing is thermally
treated under an inert gas atmosphere at a temperature of 600 °C or higher, and/or
that the process further comprises, after sintering the molding under an inert gas
atmosphere in a temperature range of 1,000 to 1,200 °C, gradually cooling the sinter
from the sintered temperature to a temperature range of 600 to 900 °C, followed by
quenching. In the process, a part of the raw material of C may be added during melting,
and the rest may be added during the pulverizing of the alloy.
[0027] The magnet according to the present invention is described in further detail below
by making reference to representative examples.
EXAMPLE 1
[0028] An alloy having the composition below was produced by a process described hereinafter.
[Chemical composition of the alloy (at.%)] |
C |
5.0 at.% |
B |
1.8 at.% |
Co |
12.0 at.% |
Nd |
13.0 at.% |
Dy |
2.5 at.% |
Tb |
0.5 at.% |
Fe |
65.2 at.% |
Where, C and B in total account for 6.8 at.%, Dy and Tb in total account for 3.0
at.%, and the ratio of Tb/Dy is 0.2.
[Production Process]
[0029] Each of the raw materials weighed as such to yield the desired alloy composition
above were molten in a vacuum melting furnace. A part of the raw material for C was
not fed into the melting furnace but was reserved. The melt thus obtained was quench
cast in a water-cooled copper casting mold from 1,600 °C to obtain a cast alloy ingot.
After heat treating under gaseous Ar at a temperature shown in Table 1, or without
applying the heat treatment, the cast alloy ingot was coarsely crushed by using a
jaw crusher, and the coarsely crushed product was fed into a vibration ball mill together
with the reserved rest of the raw material for C to perform milling. Thus was obtained
a powder having an average particle diameter of 5 µm.
[0030] The powder product thus obtained was molded under a magnetic field by applying a
pressure of 2 t/cm
2 and an external magnetic field of 15 KOe. The resulting molding was sintered under
gaseous Ar at 1,100 °C for a duration of 2 hours, and was gradually cooled from the
sintering temperature to the temperature of initiating quenching shown in Table 1,
at which temperature rapid cooling was started at a cooling rate also given in Table
1 by blowing gaseous Ar to the molding. The magnetic properties, heat resistance,
and oxidation resistance of the resulting sinter were evaluated to obtain the results
given in Table 1. The heat resistance and the oxidation resistance of the sinter were
evaluated as follows.
[Evaluation of heat resistance]
(1) Measurement of irreversible demagnetization at 200 °C
[0031] The specimen was shaped in such a manner that the permeance coefficient (Pc) thereof
be 1. More specifically, the specimen was cut out to a size of 2.5 mm × 2.5 mm × 1.05
mm.
[0032] The specimen thus obtained was magnetized by applying an external magnetic field
of 50 KOe to measure the flux value at room temperature (25 °C). A flux meter manufactured
by Toyo Jiki Kogyo Co. Ltd. equipped with an iron core coil was used to obtain the
flux value. The flux value thus obtained was designated A
25.
[0033] The magnetized specimen thus obtained was maintained at 200 °C for a duration of
120 minutes. The heating held for a duration of 120 minutes was carried out in an
oil bath filled with silicone oil. The temperature of the oil bath was precisely controlled
so that the fluctuation in temperature may fall within a range of ± 0.1 °C. The specimen
taken out from the oil bath was cooled sufficiently at room temperature to measure
the flux value again by using the flux meter above. The flux value thus obtained was
designated A
200. The irreversible demagnetization was calculated by using the observed A
25 and A
200 in accordance with the following equation:

(2) Measurement of irreversible demagnetization at 160 °C
[0034] The same procedure as that described in the measurement of irreversible demagnetization
at 200 °C above was followed to obtain observed values A
25 and A
160, except for shaping the specimen in such a manner that the permeance coefficient
(Pc) be 3 similar to the specimen described in the example of Japanese Patent Public
Disclosure No. 4-116144, and for heating the specimen in the oil bath at 160 °C for
a duration of 120 minutes. Thus, irreversible demagnetization was calculated according
to the equation above.
(3) Magnetization measurements and the temperature coefficient of coercive force
[0035] After magnetizing the specimen by applying an external magnetic field of 50 KOe,
the magnetization measurements at room temperature (RT; 25 °C) were conducted by using
a vibrating-sample magnetometer. The temperature coefficient of coercive force was
calculated in accordance with the following equation:

where, B
0 is the coercive force at room temperature, and B
1 is the coercive force obtained at 160 °C by using the same vibrating-sample magnetometer.
(4) Measurement of oxidation resistance
[0036] The progressive formation of rust was measured by performing pressure cooker test
(PCT). More specifically, the specimen was held in a testing chamber manufactured
by Tabai Espec Corp. at 120 °C, 2 atm, and 100 % RH (saturated condition) for a duration
of 100 hours, and the generation of rust was visually observed.

[0037] As shown in the results of Table 1 above, permanent magnet alloys having an irreversible
demagnetization (200 °C) of -3 % were obtained (see, for example, Table 1a). Referring
to Table 1, the irreversible demagnetization (160 °C) for alloy a is -0.7 %, a value
very close to 0 %. Thus, it can be understood that a high magnetic force is achieved
for the alloy even when used at high temperatures.
[0038] With respect to the conditions of production, it can be clearly seen by comparing
the alloy a with the alloy b that the irreversible demagnetization can be lowered
by performing heat treatment on the cast ingot. Furthermore, by comparing the results
for alloys a, c and d, the coercive force can be improved and the irreversible demagnetization
can be lowered by quenching the sintered alloy from a temperature of at least 700
°C or higher.
EXAMPLES 2 TO 16 AND COMPARATIVE EXAMPLES 1 TO 6
[0039] Sinters were prepared under the same production conditions as those employed for
alloy a in example 1, except for changing the composition of the alloys as shown in
Table 2. The characteristics of the thus obtained sintered magnets were obtained in
the same manner as described in example 1, and the results were given in Table 2.
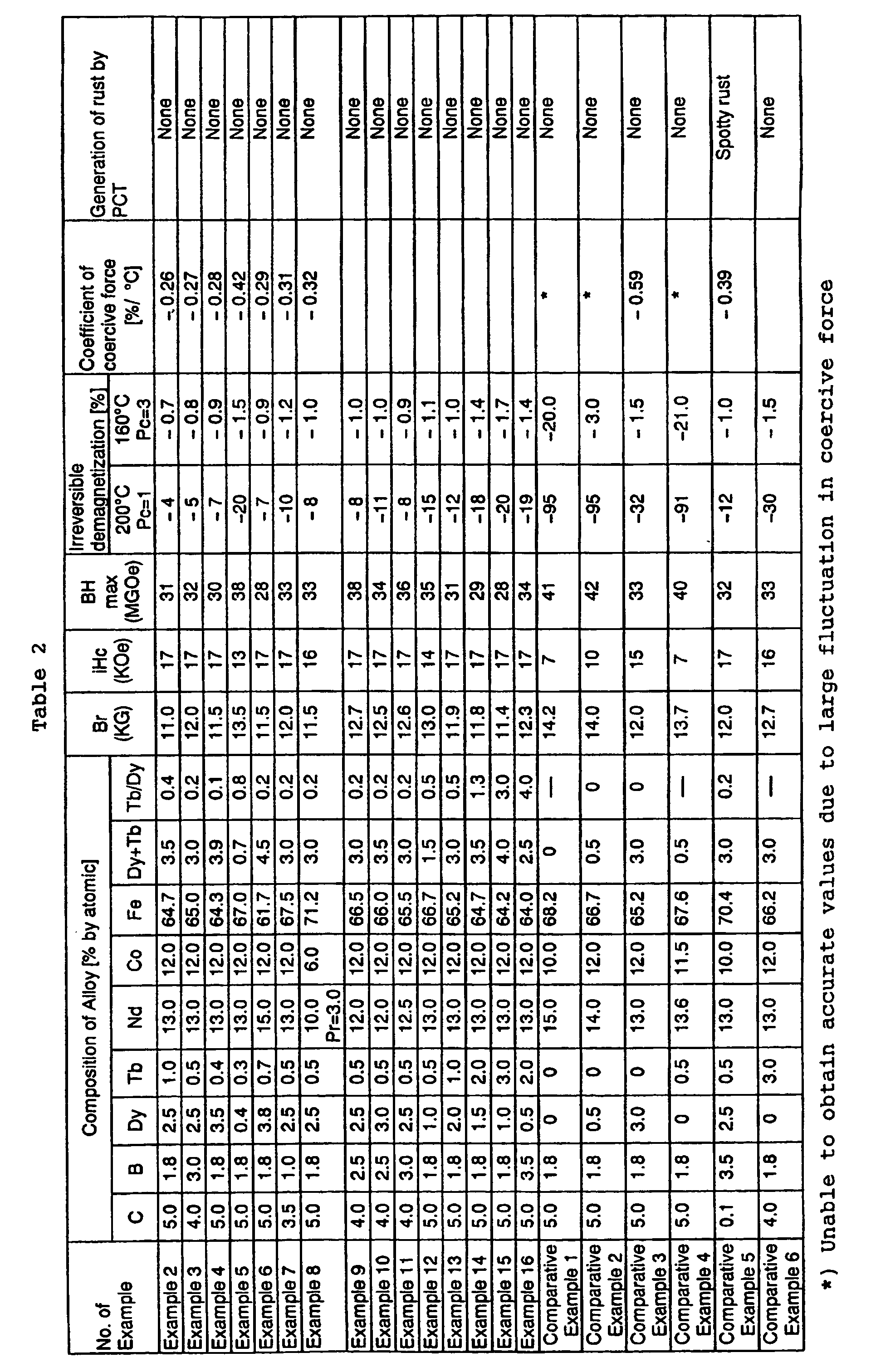
[0040] As shown in Table 3, the permanent magnet alloys of Examples 2 to 16 in which both
Dy and Tb are added all yield a low irreversible demagnetization at 200 °C, and the
irreversible demagnetization at 160 °C also is very close to 0 %. Furthermore, it
can be seen that they not only have a low temperature coefficient of coercive force,
but also exhibit excellent oxidation resistance.
[0041] In contrast to the permanent magnet alloys of the examples above, the irreversible
demagnetization at 200 °C for Comparative Example 1, in which no Dy nor Tb is added,
is as large as -95 %, that for Comparative Example 2 containing no Tb and 0.5 at.%
Dy is -95 %, and that for Comparative Example 4 containing no Dy and 0.5% by atomic
Tb is -91 %. It can be understood therefrom that these permanent magnet alloys completely
lose their magnetic force when heated to 200 °C. That is, the addition of Dy alone
or Tb alone has no effect on the irreversible demagnetization at 200 °C. Although
the addition of Dy alone in a large amount, as shown in Comparative Example 3, lowers
the irreversible demagnetization to some extent, the effect is not sufficient. Referring
to Comparative Example 5, the oxidation resistance is inferior because the content
of C falls lower than the range specified in the present invention. The alloy of Comparative
Example 6 contains 3.0 at.% of Tb but no Dy. It can be seen that, although a favorable
heat resistance is obtained, the irreversible demagnetization at 200 °C is as low
as -30 %.
[0042] Fig. 1 is a diagram showing how the values of irreversible demagnetization at 200
°C are influenced by the contents of Dy and Tb; all of the magnets given in Table
2 are plotted in the diagram wherein the abscissa shows the content of Dy (at.%) and
the ordinate shows the content of Tb (at. %). The numerals provided in Fig. 1 each
show the irreversible demagnetization at 200 °C for the plotted values.
[0043] Referring to Fig. 1, it can be understood that a peak (a point closest to 0 %) in
irreversible demagnetization at 200 °C is present for the area defined by 2 to 3 at.%
Dy and 0.3 to 1.5 at.% Tb. More specifically, the straight lines indicated by numerals
(1), (2), (3), (4), (5) and (6) define the areas with particular content of Dy and
Tb, and the area which yield an irreversible demagnetization at 200 °C in the range
of 0 to -20 % can be defined by the crossing points A, B, C and D. Similarly, the
area which yield an irreversible demagnetization at 200 °C in a range of 0 to -15
% can be defined by the crossing points B, C, H, E, F and G. The straight lines (1)
to (6) can be expressed by the following equations:






The coordinates (Dy at.%, Tb at.%) of the points A to H are obtained as follows:
Point A (0.3, 4.7)
Point B (0.3, 0.2)
Point C (0.4, 0.1)
Point D (4.9, 0.1)
Point E (4.5, 0.5)
Point F (2.8, 2,2)
Point G (0.3, 0.24)
Point H (1.0, 0.1)
[0044] Referring to Figs. 2 and 3, Fig. 2 shows the observed irreversible demagnetization
values at different temperatures for the magnet having the highest heat resistance
among the magnets disclosed in Japanese Patent Public Disclosure No. 4-116144 as described
in Example 24 thereof and those of Example 2 according to the present invention, in
which the specimens are each shaped as such that the permeance coefficient (Pc) be
3 and magnetized by applying an external magnetic field of 50 KOe. Similarly, Fig.
3 is a diagram showing the observed irreversible demagnetization values similar to
those shown in Fig. 2, except that the specimens are shaped as such that they may
yield a permeance coefficient (Pc) of 1. The magnet of Example 24 disclosed in Japanese
Patent Public Disclosure No. 4-116144 (referred to hereinafter as "disclosed magnet")
has a composition expressed by 9Nd-9Dy-59Fe-15Co-1B-7C and an irreversible demagnetization
at 160 °C at Pc = 3, according to the disclosure.
[0045] Referring to Fig. 2, the values of irreversible demagnetization at 160 °C for the
specimens shaped as such to yield Pc = 3 are -1.0 % (disclosed magnet) and -0.7 %
(Example 2 of the present invention); i.e., the difference between them is not so
large. Concerning the values of irreversible demagnetization at 200 °C, however, the
value of the magnet according to Example 2 of the present invention is improved to
-1.9 %, which can be contrasted to the value of -12.9 % for the disclosed magnet.
This tendency can be more clearly observed on the specimens shaped to yield Pc = 1,
as shown in Fig. 3. More specifically, at Pc = 1, the values of irreversible demagnetization
at 160 °C for the disclosed magnet is -9.4 %, whereas that for the magnet according
to Example 2 of the present invention is improved to -1.7 %; moreover, the values
of irreversible demagnetization at 200 °C for the disclosed magnet is -22.3 %, whereas
that for the magnet according to Example 2 of the present invention is far improved
to -4 %.
[0046] As described in detail above, the present invention provides a permanent magnet alloy
having superior heat resistance and oxidation resistance never achieved in the field
of R-Fe(Co)-B based magnets. Accordingly, the present invention provides materials
having excellent magnetic properties at low cost, which can be advantageously assembled
in appliances usable at elevated temperatures.
1. A permanent magnet alloy having an improved heat resistance comprising, in terms of
percent by atom (at.%):
0.1 to 15 at.% C
0.5 to 15 at.% B,
provided that C and B in total account for 2 to 30 at.%;
40 at.% or less Co (exclusive of zero percent),
0.3 to 4.9 at.% Dy,
0.1 to 4.7 at.% Tb,
provided that Dy and Tb in total account for 0.5 to 5 at.%;
8 to 20 at.% R,
where R represents at least one element selected from the group consisting of Nd,
Pr, Ce, La, Y, Gd, Ho, Er, and Tm;
with the balance being Fe and unavoidable incidental impurities;
wherein the irreversible demagnetization (at 200 °C) according to the following equation
(1) is in the range of 0% to -20%, where iHc is 13 KOe or higher:

where,
A
25 represents a flux value of a magnet measured at room temperature (25 °C), on a specimen
prepared into a shape such that its permeance coefficient Pc be 1 and magnetized at
50 KOe; and
A
200 represents a flux value of a magnet measured on the same specimen subjected to the
measurement of A
25, which was maintained at 200 °C for 120 minutes and then cooled to room temperature
(25 °C), for the measurement.
2. A permanent magnet alloy according to claim 1, wherein the content (at.%) of Dy and
Tb in total fall in the range defined by the points B, C, H, E, F, and G plotted in
Fig. 1, and the irreversible demagnetization at 200 °C is in the range of 0% to -15%.
3. A permanent magnet alloy according to claim 1, wherein the irreversible demagnetization
(at 200 °C) is in the range of 0% to -5%.
4. A permanent magnet alloy having an improved heat resistance according to claim 1 wherein
the ratio Tb(at.%)/Dy( at.%) is in a range of from 0.1 to 0.8.
5. A permanent magnet alloy having an improved heat resistance according to any of claims
1 to 4 wherein the content of C is in the range of 1 to 10 at.%.
6. A permanent magnet alloy having an improved heat resistance according to any of claims
1 to 5, wherein R is Nd alone or a combination of Nd and Pr.
7. A process for producing a permanent magnet alloy having an improved heat resistance
according to any of the preceding claims, said process comprising the steps of: melting
and casting each of the raw materials of the alloying elements, subjecting the resulting
alloy to pulverizing, compression molding the resulting powder, and sintering the
molding under an inert gas atmosphere in a temperature range of 1,000 to 1, 200 °C
to obtain a sintered magnet alloy characterized in that the alloy before pulverizing is thermally treated under an inert gas atmosphere at
a temperature of 600 °C or higher.
8. A process for producing a permanent magnet alloy according to claim 7 wherein the
process further comprises, after sintering the molding under an inert gas atmosphere
in a temperature range of 1,000 to 1,200 °C, gradually cooling the sinter from the
sintered temperature to a temperature range of 600 to 900 °C, followed by quenching.
9. A process for producing a permanent magnet alloy according to claim 7 or 8 wherein
a part of the raw material of C is added during melting, and the rest is added during
the pulverizing of the alloy.
1. Eine Permanentmagnetlegierung mit verbessertem Wärmewiderstand, die folgendes in Atomprozent
(at.%) aufweist:
0,1 bis 15 at.% C,
0,5 bis 15 at.% B,
vorausgesetzt, dass C and B insgesamt 2 bis 30 at.% umfassen;
40 at.% oder weniger Co (ausschließlich Null Prozent),
0,3 bis 4,9 at.% Dy,
0,1 bis 4,7 at.% Tb,
vorausgesetzt, dass Dy und Tb in einer Gesamtmenge von 0,5 bis 5 at.% vorhanden sind;
8 bis 20 at.% R,
wobei R mindestens ein Element repräsentiert, welches aus der aus folgendem bestehenden
Gruppe ausgewählt ist: Nd, Pr, Ce, La, Y, Gd, Ho, Er und Tm;
wobei der Rest Fe und nichtvermeidbare zufällige Verunreinigungen sind;
wobei ferner die irreversible Entmagnetisierung (bei 200°C) gemäß der folgenden Gleichung
(1) im Bereich von 0% bis -20% liegt, wobei iHc 13 KOe oder höher ist:

wobei folgendes gilt:
A25 repräsentiert einen Flusswert eines Magneten gemessen bei Raumtemperatur (25°C) an
einer Probe hergestellt in einer Form derart, dass deren Permeanzkoeffizient Pc 1
ist und zwar magnetisiert bei 50 KOe; und
A200 repräsentiert einen Flusswert eines Magneten gemessen an der gleichen der Messung
von A25 ausgesetzten Probe, wobei die Probe auf 200°C 120 Minuten lang gehalten wurde und
sodann auf Raumtemperatur (25°C) für die Messung abgekühlt wurde.
2. Permanentmagnetlegierung nach Anspruch 1, wobei der Gehalt (at.%) von Dy und Tb insgesamt
in den durch die Punkte B, C, H, E, F und G gemäß Fig. 1 definierten Bereich fallen
und wobei die irreversible Entmagnetisierung bei 200°C im Bereich von 0% bis -15%
liegt.
3. Permanentmagnetlegierung nach Anspruch 1, wobei die irreversible Entmagnetisierung
(bei 200 °C) in einem Bereich von 0% bis -5% liegt.
4. Permanentmagnetlegierung mit einem verbesserten Wärmewiderstand gemäß Anspruch 1,
wobei das Verhältnis von Tb (at.%) / Dy (at.%) im Bereich von 0,1 bis 0,8 liegt.
5. Permanentmagnetlegierung mit einem verbesserten Wärmewiderstand nach einem der Ansprüche
1 bis 4 , wobei der Gehalt an C im Bereich von 1 bis 10 at.% liegt.
6. Permanentmagnetlegierung mit einem verbesserten Wärmewiderstand nach einem der Ansprüche
1 bis 5, wobei R allein Nd oder eine Kombination von Nd und Pr ist.
7. Ein Verfahren zur Herstellung einer Permanentmagnetlegierung mit einem verbesserten
Wärmewiderstand gemäß einem der vorhergehenden Ansprüche, wobei das Verfahren die
folgenden Schritte vorsieht: Schmelzen und Gießen jedes der Rohmaterialien der Legierungselemente,
Aussetzen der sich ergebenden Legierung gegenüber einer Pulverisierung, Kompressionsformung
des sich ergebenden Pulvers und Sintern des Formlings unter einer in InertGasatmosphäre
in einem Temperaturbereich von 1000 bis 1200°C , um eine gesinterte Magnetlegierung
zu erhalten,
dadurch gekennzeichnet, dass die Legierung vor der Pulverisierung thermisch in einer inerten Gasatmosphäre bei
einer Temperatur von 600°C oder höher behandelt wird.
8. Verfahren zur Herstellung einer Permanentmagnetlegierung gemäß Anspruch 7, wobei das
Verfahren ferner folgendes aufweist: nach dem Sintern des Formlings unter einer inerten
Gasatmosphäre in einem Temperaturbereich von 1000 bis 1200°C allmähliches Abkühlen
des gesinterten Körpers von der Sintertemperatur auf einen Temperaturbereich von 600
bis 900°C, gefolgt von einem Abkühlvorgang.
9. Verfahren zur Herstellung einer Permanentmagnetlegierung gemäß Anspruch 7 oder 8,
wobei ein Teil des Rohmaterials C während des Schmelzens zugegeben wird und der Rest
während der Pulverisierung der Legierung zugegeben wird.
1. Alliage d'aimant permanent à résistance à la chaleur améliorée comprenant, en atomes
pourcent (at.%) :
0,1 à 15 at.% de C,
0,5 à 15 at.% de B,
pourvu que C et B ensemble forment de 2 à 30 at. % ;
40 at.% ou moins de Co (mais pas zéro pourcent),
0,3 à 4,9 at.% de Dy,
0,1 à 4,7 at.% de Tb,
pourvu que Dy et Tb forment ensemble de 0,5 à 5 at. % ;
de 8 à 20 at.% de R,
où R représente au moins un élément choisi dans le groupe comprenant Nd, Pr, Ce,
La, Y, Gd, Ho, Er et Tm ;
le reste étant du fer et des impuretés accidentelles inévitables ;
dans lequel la désaimantation irréversible (à 200°C) selon l'équation (1) suivante
est dans la plage de 0 à -20 %, où iHc est de 13 kOe ou plus :

où,
A
25 représente une valeur de flux d'un aimant mesurée à température ambiante (25°C) sur
un spécimen préparé selon une forme telle que son coefficient de perméance Pc est
de 1 et aimanté à 50 kOe ; et
A
200 représente une valeur de flux d'un aimant mesurée sur le même spécimen soumis à la
mesure de A
25 qui a été maintenu à 200°C pendant 120 minutes puis refroidi à température ambiante
(25°C) pendant la mesure.
2. Alliage d'aimant permanent selon la revendication 1, dans lequel le contenu total
(at.%) de Dy et Tb tombe dans la plage définie par les points B, C, H, E, F et G représentés
en figure 1, et la désaimantation irréversible à 200°C est dans la plage de 0 à -15
%.
3. Alliage d'aimant permanent selon la revendication 1, dans lequel la désaimantation
irréversible (à 200°C) est dans la plage de 0 % à -5 %.
4. Alliage d'aimant permanent à résistance à la chaleur améliorée selon la revendication
1, dans lequel le rapport Tb (at.%)/Dy (at.%) est dans la plage de 0,1 à 0,8.
5. Alliage d'aimant permanent à résistance à la chaleur améliorée selon l'une quelconque
des revendications 1 à 4, dans lequel le contenu en C est dans la plage de 1 à 10
at.%.
6. Alliage d'aimant permanent à résistance à la chaleur améliorée selon l'une quelconque
des revendications 1 à 5, dans lequel R est Nd seul ou une combinaison de Nd et Pr.
7. Procédé de fabrication d'un alliage d'aimant permanent à résistance à la chaleur améliorée
selon l'une quelconque des revendications précédentes, ce procédé comprenant les étapes
consistant à fondre et mouler chacun des matériaux bruts des éléments d'alliage, soumettre
l'alliage résultant à une pulvérisation, mouler sous pression la poudre résultante
et fritter le moulage sous une atmosphère de gaz inerte dans une plage de température
de 1000 à 1200 °C pour obtenir un alliage d'aimant fritté, caractérisé en ce que l'alliage avant pulvérisation est traité thermiquement dans une atmosphère de gaz
inerte à une température supérieure ou égale à 600°C.
8. Procédé de formation d'un alliage d'aimant permanent selon la revendication 7, comprenant
en outre, après frittage du moulage dans une atmosphère de gaz inerte dans une plage
de température de 1000 à 1200°C, un refroidissement progressif de la fritte depuis
la température de frittage jusqu'à une plage de température de 600 à 900°C, suivi
d'une trempe.
9. Procédé de fabrication d'un alliage d'aimant permanent selon la revendication 7 ou
8, dans lequel une partie de la matière brute de C est ajoutée pendant la fusion et
le reste est ajouté pendant la pulvérisation de l'alliage.