[0001] The invention relates to a method of drafting and building a prestressed concrete
structure, especially in the field of concrete bridge engineering. The invention further
relates to a prestressed concrete structure.
[0002] Adequate drafts of prestressed concrete bridges have to fulfill all the prestressing
requirements, which are to be met for a prestressed cross section as optimally as
possible along the entire length of the structure. Due to the fact that the parameters
of prestressing, such as the run and force of prestressing strands in a section, affect
the stresses in all other sections, an improvement of the draft of prestressing of
concrete bridges can, up until now, only be performed on the basis of iteration and
the experienced data of the drafting engineer. If there is no success with reference
to a constant quality of the draft over the entire length of the structure, there
are excessive compressive stresses and, as the case may be, likewise adverse tensile
stresses distributed over wide ranges of the structure as well as a higher demand
for the total amount of prestressing steel.
[0003] WO 01/27406 A1 discloses a method for designing and fabricating a multistep tension prestressed
girder. According to this method, prestress is introduced with respect to a relationship
between a load and stress, for each step of construction, so that the height of a
profile of the girder can be reduced.
[0005] Accordingly, there is a demand for an elaborate, iterative search for an optimal
prestressing, especially within statically indeterminate load-bearing systems of sectionably-erected
bridges. Therefore, it is an object of the invention to provide an improved method
of drafting concrete elements with optimum prestressing, especially in the field of
concrete bridge engineering, that can be performed by the use of computers.
[0006] According to the invention, the above object is met by a method of drafting a prestressed
concrete structure comprising the features of claim 1. Advantageous and expedient
embodiments of the invention are given in the dependent claims.
[0007] The method of drafting and building a prestressed concrete structure according to
the invention comprises the steps of:
- a) modeling the problem of finding optimum parameters of prestressing strands in the
prestressed concrete structure in a mathematical optimization problem;
- b) using a preferably gradient-based numerical search method to solve the mathematical
optimization problem; and
- c) building the prestressed concrete structure in accordance with the results of step
b).
[0008] The method according to the invention links technical engineering knowledge with
numerical search methods in a unique manner. By the use of the method according to
the invention, the draft of the prestressing of concrete bridges can be carried out
by the use of a computer; and thereby, the optimal distribution of prestressing forces
and the optimal run of strands and tendons, respectively, within the relevant span-section
can be found in order to avoid excessive compressive stresses and the occurrence of
adverse tensile stresses under the considered load-level over the entire length of
the structure.
[0009] Thus, the invention provides, for the first time, a computerizable procedure for
the draft of the prestressing of concrete bridges based on non-linear numerical methods
for optimization. Hereby, the prestressing force required for each section, as well
as the run of the prestressing strands with reference to their length, elevation within
the cross section and curvature, can be found and optimized.
[0010] The primary criterion of optimization is to achieve a minimum of the total amount
of the prestressing steel necessary. Thereby, an improvement of the overall draft
is achieved since excessive compressive stresses as well as adverse tensile stresses,
as often seen in the case of a non-optimized draft of prestressing over wide ranges
of the structure, are avoided.
[0011] Besides the economic advantages of an optimized prestressing, there are, above all,
additional advantages concerning the draft of the construction. Those include more
balanced and lower average concrete stresses compared to the results of a draft of
the prestressing done "by hand". Therefore, a lower creep-induced loss of prestressing
forces is to be considered; and, the probability of the appearance of cracks is reduced.
[0012] Another benefit is that due to the fact that the draft of prestressing strongly integrates
the effects of the statically indeterminate prestressing-induced bending moments,
there is a reduced susceptibility of the load-bearing structure against irregularities
concerning dimension accuracy. This is due to the fact that the integral value of
statically indeterminate bending moments is relatively stable with respect to possible
local irregularities such as scattered discrepancies of installation dimensions with
regard to the prestressing tendons.
[0013] It is expected that by means of the proposed method, harmonic, economic and statically
useful prestressing parameters will be achieved for real applications, which fulfill
all prestressing requirements in the best possible way along the entire length of
the structures concerned.
[0014] According to the invention, in above-mentioned step a) of the method a constrained
non-linear optimization problem is modeled, including an objective function to be
optimized, equality constraints and inequality constraints.
[0015] According to the invention, the mechanical equations used for the objective function
and/or for the equality and/or inequality constraints are formulated based on principles
of virtual work, instead of using finite element data. The parameters of prestress
are themselves introduced as unknown quantities into the principle of virtual forces.
In fact, the complicated involvement of a finite element method has prevented civil
engineers from using a mathematical optimization method for drafting prestressed concrete
structures to date, especially in the field of concrete bridge engineering. By contrast,
virtual work principles can be embedded into an optimization procedure much easier
than a finite element method. The use of virtual work principles leads to a single
(albeit very large) optimization equation capturing all relevant constraints. Since
this equation is continuous enough and therefore differentiable at each point, any
common gradient-based fast numerical search method can be utilized. This makes the
method according to the invention both variable and fast.
[0016] Details of the invention will become apparent from the following description and
from the accompanying drawings to which reference is made. In the drawings:
- Figure 1 shows a fictional construction-section 1 divided into sections 1 to i for
the formulation of the mechanical equations;
- Figure 2 shows known prestressing-induced virtual moments and unknown elevation of
strands and statically determinate prestressing-induced moments;
- Figure 3 shows a range for strands with variable and fixed anchoring points;
- Figure 4 shows a possible scheme of strands with variable and set points of anchorage
fixture;
- Figure 5 shows the run of strands referring to the center of gravity of the superstructure's
cross section;
- Figure 6 shows optimized lengths and forces of prestressing strands referring to Table
1; and
- Figure 7 shows statically indeterminate prestressing-induced bending moments (dimensionless).
I. PRINCIPLES OF OPTIMIZATION
I.1 GUIDING PRINCIPLE OF OPTIMIZATION
[0017] In the course of finding solutions within the scope of engineering and applied sciences,
often minimal or maximal values of results are to be found with the least amount of
effort in calculation. The search for an extreme value under consideration of the
given boundary conditions and constraints represents the essential guiding principle
of optimization.
1.2 PROCEDURE OF OPTIMIZATION
[0018] Since there are only few analytical solutions possible for optimization problems,
the mathematical calculation is mostly based on numerical search methods. Such methods
are distinguished into gradient-based methods on the one side, and direct methods
on the other side (see
Gekeler, E.W., "Mathematische Methoden zur Mechanik," Springer Verlag, December 2006, and
Alt,W., "Nichtlineare Optimierung," Vieweg Verlag, May 2002).
[0019] In order to solve the problem at hand, a gradient-based method was chosen since the
advantages of the direct methods could not be utilized and the underlying objective
function, which is described in more detail in chapter 11.3, is differentiable within
the whole domain and therefore, the gradient detectable. Especially due to the high
number of unknowns, the so-called "Interior Point Method" (see
Vanderbei, R.J., "An interior point code for quadratic programming," Technical Report
SQR 94-15, Princeton University, 1994, and
Vanderbei, R.J., and Shanno, D., "An interior point algorithm for nonconvex nonlinear
programming," Technical Report SQR 97-21, Princeton University, 1999) is referred to.
[0020] It should be noted that, in general, the mentioned procedures are only able to detect
local extremes. However, by the use of a suitable starting point variation, the probability
of finding a global extreme is increased and even guaranteed in individual cases.
[0021] The interior point algorithm solves a constrained optimization by combining constraints
and the objective function f(x) through the use of a barrier-function,
qkB(
x) (see
Bronstein, I.N., Semendjajew, K.A., Musiol, G., and Mühlig, H., "Taschenbuch der Mathematik,"
Harri Deutsch Verlag, June 2000). The function guarantees that the solution lies within the feasible area. The barrier-function
B(x), with the additional parameter q
k, becomes larger the closer it is to the edge of an admissible area so that the barriers
of this area cannot be overstepped.
[0022] Specifically, the general constrained optimization problem is first converted to
the standard form of a non-linear optimization problem:

[0023] The objective function f(x) represents the objective function which is to be optimized
depending on the unknown vector x; h(
x) represents the equality constraints and g(
x) the inequality constraints.
[0024] The inequality constraints are replaced by a system of equalities in order to avoid
an inequality system.
[0025] With the necessary Karush-Kuhn-Tucker condition (equation 3) we get a system of non-linear
equations.

[0026] The vector
yT describes the langrangian multipliers; and, the matrix
A (x) consists of the partial gradients of the equality constraints
h(
x).
[0027] The system can then be solved by standard algorithms for non-linear systems of equations,
where the "Newton Method" or "Newton Algorithm" (see
Bronstein, I.N., Semendjajew, K.A., Musiol, G., and Mühlig, H., "Taschenbuch der Mathematik,"
Harri Deutsch Verlag, June 2000, and
Rade, L., and Westergren, B., "Mathematische Formeln," Springer Verlag, April 2000) is a proved, stable and often used solver. It is also possible to use standard gradient
algorithms; however, there is one important drawback. Using the standard algorithms,
it is important that the start-vector for the search of the optimum lies within the
feasible domain.
II. APPLYING OPTIMIZATION TO THE DRAFT OF PRESTRESSING OF CONCRETE BRIDGES
11.1 PRINCIPLE STRATEGY AND GOALS OF OPTIMIZATION
[0028] In order to formulate the task of optimization, all mechanical equations needed for
the objective function as well as for the equality and inequality constraints are
to be formed and transformed into a system which is computationally manageable.
[0029] Those equations should be formulated directly based on the principles of virtual
work. This is more variable and requires less time for calculation due to their small
amount of data volume compared to the finite element method. Hereby, for example,
the consideration of the effects due to cracked concrete would even be possible if
the relevant moment-curvature-relation is known for a section.

[0030] The virtual operational equations can be formulated for the bending girder proceeding
from continuum according to W
int in equation 5. By comparing internal and external work-values, deformations on the
basis of real and virtual bending moments can be calculated.
[0031] Statically indeterminate load-bearing systems are first to be transformed into statically
determinate systems through the introduction of virtual hinges. Proceeding from the
compatibility condition for continuous beams, after which the bending line has no
kinks, the basic equations for the optimization of statically indeterminate systems
are developed. The resulting virtual back-twisting bending moments correlate to the
statically indeterminates.
[0032] The goal of the optimization is to find the optimal relevant prestressing parameters
along the entire structure. In this case, the prestressing cables act effectively
and therefore, the total amount of the necessary prestressing steel will be minimized.
During the search for an optimal prestressing, the prestressing force, run, as well
as length of the cables or strands, respectively, have to be considered as variable
according to the relevant conditions of statics.
[0033] Furthermore, the following particular conditions should be taken into account: sectionable
erection of the superstructure (where applicable); time-dependent loss of prestressing
forces due to creep and shrinkage of concrete; primary constraints regulated by national
codes, such as the limitation of longitudinal, compressive and tensile stresses under
defined load-levels; friction-induced loss of prestressing force along the cables;
and, the consideration of uncoupled, continuous cables along wide ranges of the structure.
In part, such as in the sectionable erection of superstructures, this can already
be taken into account within the formulation of the principle mechanical equations.
[0034] Further conditions, such as, for example, the limitation of the longitudinal stresses
due to bending moments and axial forces, have to be formulated as inequality constraints
limiting the possible range of solutions.
11.2 FORMULATION OF THE OPTIMIZATION EQUATIONS
[0035] The general strategy is shown in more detail in the following by way of an example
of a fictional 3-span statically indeterminate bridge superstructure which is considered
as to be erected in two sections due to economic reasons.
[0036] In the case of prestressed concrete bridges, all primary parameters of prestressing
depend on the load-induced bending moments and axial forces as well as on prestressing-induced
bending-moments themselves. The basic equation can therefore be derived from the course
of bending moments and axial forces resulting from load and prestressing effects taking
into account all additional effects which are to be considered.
[0037] The statically determinate and the statically indeterminate parts of prestressing,
as well as the time-dependent variation of bending moments due to the different age
of the concrete within the sequential sections of erection, should be included in
that basic equation.
[0038] For the numerical description, the superstructure has to be divided into small subsequent
sections. By way of example,
Figure 1 shows a fictional construction-section 1 divided into sections 1 to i for the formulation
of the mechanical equations.
[0039] After that, the mechanical properties of the cross section are assigned for the relevant
sections of the superstructure. The force of the relevant prestressing strands and
the strand's elevation within the cross section correlate to that system as well.
[0040] The statically determinate moment-parts,
x[
i] ·
ajk[
i] · σ
0[
i], as well as the statically indeterminate moment-parts, Mj[i], are represented by
a vector of length k, where j is the order of statically indeterminacy. k corresponds
to the number of sections introduced.
Figure 2 shows the known prestressing-induced virtual moments (left) and the unknown elevation
of strands and the statically determinate prestressing-induced moments, respectively
(right)
[0041] The statically determinate (real) parts of the prestressing moments cause twistings
Δ
10 and Δ
20 within the integrated virtual hinges. Δ
10 here corresponds to the real twisting at the virtual hinges due to the prestressing.
Due to compatible conditions, those must be compensated by the statically indeterminate
twisting parts resulting from the virtual bending moments Δ
11, Δ
22, Δ
12 and Δ
21. The value Δ
11 hereby corresponds to the back-twisting at the first interior column due to the virtual
bending moments M1 with the normalized value 1; and, Δ
12 corresponds to the back-twisting at the first interior column due to the virtual
bending moment M2.
[0042] The integrals in equation 5 are calculated in two steps with respect to a more effective
numerical handling. First, the related statically determinate moments proceeding from
the prestressing are calculated, resulting in the form of a trapezoid-shaped area
(see
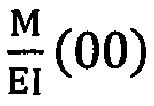
in
Figure 2 on the right).
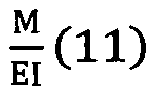
coresponds to the effects of the statically-indeterminate bending moment M1 at the
first interior column.
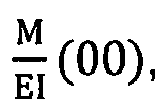
as well as the virtual related parts of bending moments
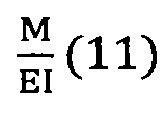
and

are described by a vector with a reduced length of k-1 since, instead of the sections,
here only small area-parts are used. Incidentally, this is the first part of the integration
procedure. It is mentioned that the vectors for the statically indeterminate parts
of the prestressing already include all unknowns such as the length x(i) of the strands
as well as the amount a
jj[i] or the force, respectively, of the appropriate prestressing strand.
[0043] The vectorial description leads to a very simple formulation of a global equation
for the prestressing-induced bending moments under the consideration of all conditions.
The indices always refer to the part of the superstructure being considered or active.

[0044] For the case in which there is not a sectionable erection of the superstructure,
the statically indeterminate parts of the prestressing over the interior columns can
already be calculated depending on the unknown elevation x[i] of the strand within
the cross section and the unknown amount of prestressing force a
jk[i] by the use of a simple matrix scheme. The multiplication of vectors here also
corresponds to the formulation of integrals necessary within the principle of virtual
works.
[0045] Through the internal product of the vectors, the second step of the numerical integration
procedure of the integrals in (5) is carried out. It should, however, be mentioned
that its scaling is performed by 1/EI; this is, however, simplified in the course
of the matrix multiplication. As a result, there is an equation for the determination
of prestressing-caused, statically-indeterminate bending moments over the interior
columns of the load-bearing system.

[0046] X1
end coresponds to the actual value of the bending moment, M1, and represents nothing
more than the statically indeterminate part of the prestressing-induced bending moments
over the first interior column and for X2
end correspondingly.
[0047] It should be mentioned once more that the calculated, statically indeterminate parts
X1 and X2 are now functions depending on the unknowns such as elevation x[i] and force
ajj[i] of the relevant strand-section. The multiplication of the values, X1 and X2,
respectively, with the normalized course of the bending moments, M1 and M2, respectively,
results in the run of the statically indeterminate parts for each considered hinge.
[0048] In the case of a sectionable erection, it also has to be considered that only a certain
part of the prestressing elements is active within the relevant section. Only this
part is responsible for the effect of statically indeterminate prestressing-induced
bending moments within this erected section. The time-dependent variation of bending
moments due to creep and shrinkage of the concrete can be determined, for example,
by the use of the variation-factor C
cr=(1-c
v) from Trost (see Mehlhorn, G., "Der Ingenieurbau - Bemessung", Ernst & Sohn, April
1999).
[0049] In the following section, all prestressing elements which do not fall within the
relevant section are to be considered as inactive.
[0050] By way of example,
Figure 3 shows a range for strands with variable and fixed anchoring points. In section 1
of erection only the strands a11, a21, a31, a2, and a3 are active. All others are
inactive. In the first section, a statically indeterminate part, X1
sec1, is only possible over the first interior column. Since it deals with a product of
vectors, the following equation provides values for the prestressing-induced bending
moments depending on the unknowns.

[0051] Within the construction-section 2, the additional parts over the first interior column
as well as the parts over the second interior column are to be calculated. From that,
a solution vector with the result values (X1
sec2,X2
sec2)
T can be derived.
[0052] The final statically indeterminate parts, which are to be multiplied with the triangle-functions
in
Figure 2, accrue from the superposition of the section-values with the values based on a static
system without any section joints and without section-part X1
end taking into account the above-mentioned variation-factor due to the time-dependent
variation of bending moments.
[0053] The following equation provides for the statically indeterminate prestressing-induced
bending moments over the first interior column in the considered 3-span-system.

[0054] Through a superposition of the statically determinate and statically indeterminate
parts of prestressing, a global equation can be formulated for the total prestressing-induced
bending moments depending on the unknown length, run and force of the strands.
[0055] The effects resulting from the erection procedure are fully included in that equation.
The latter is a basis for the formulation of the objective function as well as the
constraints.
[0056] Taking into account the statically indeterminate parts of the prestressing, the position
of the centers of moments, calculated on the basis of the dead-load of the bridge,
can vary marginally. Therefore, it is recommended to define the possible anchorage-points
of the strands surrounding the above-mentioned corrected centers of moments. Hereby,
the optimal effect of prestressing is made possible. However, the numerical effort
is comparably high.
11.3 FORMULATION OF THE OBJECTIVE FUNCTION AND CONSTRAINTS
[0057] The objective function as well as the constraints can now easily be formulated as
vector-equations for each relevant section. It is for example ∑a
jj[i] = min.
[0058] For instance, conditions for edge stresses, which also proceed from bending equations,
can be formulated as constraints. Likewise, relations between subsequent strands,
admissible curvatures of strands or non-negative constraints can also serve as constraints.
[0059] With the constraint after which the force of all serial strands to the right must
always be larger or equal than the relevant previous strand to the left, the right
side for the allocation of the prestressing anchorage fixture is set. This holds vice
versa as well.
[0060] The solving of the problem can be conducted by the use of the above-mentioned algorithm.
In order to assure a fast convergence of the solution-procedure, a start-vector for
the prestressing which refers, for example, to the curvature of bending moments resulting
from the dead-loads of a superstructure can be defined. The statically indeterminate
parts of the prestressing can generally be neglected in the primary approach.
III. APPLICATION BY THE USE OF AN EXAMPLE AND INTERPRETATION OF THE RESULTS
111.1 DESCRIPTION OF THE EXAMPLE AND OF THE PRESTRESSING CONCEPT
[0061] The applicability of the shown method will be demonstrated qualitatively by the way
of a prestressed sectionably-erected 6-span-highway-viaduct which is planned to be
built in Germany (see Bulicek, H., "Comparison of the Application of Internal Prestressing
Unbonded Tendons with Bonded Tendons by the example of the Schallermühle Viaduct,
Innsbrucker Bautage 2009," V. 3, iup (Innsbruck University Press), Innsbruck (Austria),
January 2009, pp. 157-168). The considered superstructure of the bridge is prestressed
in the longitudinal direction by the use of internal, post-tensioned grouted tendons.
The 240 m long structure with a multi-web T-beam cross-section will be erected in
three sections, whereby span 3 and span 5 will house the relevant section joint and
couple-points for the cables respectively. The span is approximately 32 m in the first
and last span and 44 m in the four middle spans. At least 50 % of the amount of prestressing
steel in the particular section should be run through that section (see thin line
in
Figure 4 which shows a possible scheme of strands with variable and set points of anchorage
fixture).
[0062] In general, the following decision has to be made before starting the optimization
process. As a rule, there is a pre-defined choice of the length of the individual
strands with respect to the construction process such as the possibility to set anchorage
fixtures and couplings. In this case, only the run of the relevant strands and their
force is variable with reference to the optimization. Alternatively, it is also possible
to consider the length of the strands as well as the run of the strands as being variable
(see thick lines in
Figure 4). In the following, the latter is considered.
[0063] As is seen in
Figure 3, the given section joints limit the possibility of varying the length of the strands.
In all other areas, the allocation of the anchorages is variable with respect to the
optimization process.
[0064] As already mentioned before with respect to the allocation of the prestressing anchorage
fixture, as a constraint the force of all serial strands to the right must always
be larger or equal than the relevant previous strand to the left. This holds vice
versa as well.
[0065] Concerning the iteration, the solution which requires a minimal amount of prestressing
steel is memorized for each section considered. This value is then used as a start
value for the next section. The optimal length of the strands is strongly influenced
by the statically indeterminate, prestressing-caused bending moments next to the actual
run of the strands.
III.2 RESULTS OF THE CALCULATION
[0066] For the above-mentioned superstructure, the prestressing has been drafted by the
use of this non-linear optimization procedure.
[0067] Figures 5 and 6 show the relevant results qualitatively. The run of the prestressing strands is displayed
for each section considered. The position of the center of gravity of the strands
is also allocated automatically based on a minimum total amount of prestressing steel.
However, in order to avoid prestressing strands, which are impracticably short, a
minimum length should be defined within the definition of the constraints.
[0068] The following Table 1 indicates the relative amount of prestressing force in the
relevant strands along the superstructure.
Tendon-no. |
Dimensionless amount of prestressing force |
A11, A12, A13 |
|
54 % |
A2 |
|
0 % |
|
A21 |
27 % |
A3 |
|
46 % |
|
A31 |
46 % |
A4 |
|
38 % |
|
A41 |
38 % |
A5 |
|
38 % |
|
A51 |
44 % |
A6 |
|
38 % |
|
A61 |
38 % |
A7 |
|
38 % |
[0069] Now remaining is the important task of the drafting engineer to choose the relevant
prestressing cables in order to cover the necessary amount of optimized prestressing
forces along the entire superstructure and fit them into the cross section according
to the optimized run, meanwhile taking into account the specific constraints of all
the givens.
III.3 INTERPRETATION OF RESULTS
[0070] As it is seen in the numerical results, the statically indeterminate prestressing-induced
bending moments (see
Figure 6 which relates to the values of Table 1) play an important role in the finding of
an optimal conduction of the prestressing strands.
[0071] It also shows that for an optimized run of the prestressing strands, the latter must
not always be conducted close to the edge of the cross section, depending on, of course,
the givens of the relevant case. The finding of an optimal run of the prestressing
strands "by hand", is therefore, especially for large bridges with variable cross
sections, a very time-consuming endeavor requiring much instinctive feeling on the
part of the drafting engineer with regard to the prestressing of concrete bridges.
[0072] Figure 7 shows the statically indeterminate prestressing-induced bending moments (dimensionless).
IV. SUMMARY AND OUTLOOK
[0073] In its structure, the presented method for drafting and building a prestressed concrete
structure, in particular a bridge, combines, on the one hand, engineering, constructional
and mechanical knowledge with numerical search methods, on the other hand. In doing
so, the performance is strongly influenced by several points which are to be considered
from the constructional point of view. Above all, the scheme of selecting the prestressing
strand sections (see Figure 4) is to be mentioned. This results in a reduction of
the total number of unknowns due to the fact that the amount of prestressing steel
elements is combined to prestressing strands at the individual intersections.
[0074] Generally, the structure of the presented method for drafting and building a prestressed
concrete may be subdivided into 5 segments:
- 1. Setting up a mostly non-linear system of equations combining the effect of the
prestressing with the longitudinal forces and the bending moments in the bridge structure.
Not only can the principle of virtual forces (as described before) be utilized for
this subtask as a numerical variant.
Setting up the system of equations may also be carried out using finite elements as
basic elements. In doing so, merely the effect of the prestressing has to be numerically
described through the curvature of the prestressing strands. Thus, the level of the
strands is introduced into the equation in an indirect way.
The system of equations may also be formulated for the respective prestressing strands
with the principle of the virtual forces but using spline functions (so-called "B-splines").
In doing so, each prestressing strand Ai is described as a B-spline. The form of the strand can be defined in most cases by
means of few (but at least seven) test points. All method-related numerical differentiations
and integrations are carried out then by the B-Spline functions. With this, the description
of the prestressing strand position is possible with a lower number of supporting
points whereby the numerical effort for solving the problem will crucially decrease.
- 2. Selecting a suitable, problem-related objective function. The solution behavior
is greatly impacted by the selection of the objective function. In case of using function
parts with oscillating functions courses, it may happen that a global solution cannot
be found. In the context of the presented method, the sum of the prestressing strand
areas ∑j ∑i Aij is used. This selection is only possible due to the innovative prestressing strand
scheme. This is why the solution search is carried out on the basis low-order n-dimensional
polynomials and the convergence is certain.
- 3. Formulating suitable constraints and boundary conditions which are applicable to
the respective construction progress as well as the related construction method of
the bridge structure. The constraints and boundary conditions allow considering, inter
alia, the side of force introduction, the admissible bending radii of the tendons,
friction-related losses along the respective prestressing strand as well as the normative
constraints in the method. The side of force introduction may be defined by the condition
A21(i) ≥ A20(i) ^ A31(i) ≥ A30, for example. Again, the selected prestressing strand scheme allows using this type
of boundary condition and permits a combination of the construction method with the
system of equations.
- 4. Numerical solution of the system of equations with boundary conditions. Here, classical
gradient-based as well as stochastic search methods may be used. The structure of
the problem, however, prefers the methods mentioned at first.
- 5. Evaluation of the results, and building the bridge based on the results.
1. A method of drafting and building a prestressed concrete structure, the drafting being
performed by the use of computers, the method comprising the steps of:
a) modeling the problem of finding optimum parameters of prestressing strands in the
prestressed concrete structure in a mathematical optimization problem; and
b) using a, preferably gradient-based, numerical search method to solve the mathematical
optimization problem; and
c) building the prestressed concrete structure in accordance with the results of step
b);
characterized in that in step a) a constrained non-linear optimization problem is modeled, including an
objective function to be optimized, equality constraints and inequality constraints;
the objective function preferably relating to the total amount of the necessary strands
material, in particular steel, in the prestressed concrete structure; and
in that mechanical equations used for the objective function and/or for the equality and/or
inequality constraints are formulated based on principles of virtual work, preferably
involving transforming statically indeterminate load-bearing systems into statically
determinate systems through the introduction of virtual hinges;
2. The method according to claim 1, characterized in that mechanical equations used for the objective function and/or for the equality and/or
inequality constraints are formulated based on using finite elements as basic elements,
or based on principles of virtual forces and using spline functions.
3. The method according to claim 1 or 2, characterized in that the equality constraints and/or inequality constraints relate to relevant conditions
of statics involving at least one of the following strand parameters: prestressing
force, run, length; wherein the equality constraints and/or inequality constraints
preferably further relate to at least one of the following particular conditions:
sectionable erection of the structure; time-dependent loss of prestressing forces
due to creep and shrinkage of concrete; limitation of longitudinal, compressive and
tensile stresses under defined load-levels; friction-induced loss of prestressing
force along the strands; consideration of uncoupled, continuous strands along wide
ranges of the structure; limitation of the longitudinal stresses due to bending moments
and axial forces.
4. The method according to any of the preceding claims, characterized in that step a) involves virtually dividing the structure into small subsequent sections
for numerical description and assigning mechanical properties of the cross section
for relevant sections of the structure, including the force of relevant prestressing
strands and the strands' elevation within the cross section.
5. The method according to any of the preceding claims, characterized in that formulating a basic optimization equation in the optimization problem is derived
from a course of bending moments and axial forces resulting from load and prestressing
effects, wherein preferably at least one of the following is included in the basic
equation: statically determinate and statically indeterminate parts of prestressing;
time-dependent variation of bending moments due to different age of concrete within
sequential sections of erection.
6. The method according to claim 5, characterized in that through a superposition of statically determinate and statically indeterminate parts
of prestressing, a global equation is formulated for the total prestressing-induced
bending moments depending on the unknown parameters of the strands, the global equation
being the basis for formulating the objective function to be optimized.
7. The method according to any of the preceding claims, characterized in that one of the constraints is the condition that the force of all serial strands to the
right must always be larger or equal than the relevant previous strand to the left,
and vice versa, in order to set the right side or the left side, respectively, for
an allocation of a prestressing anchorage fixture.
8. The method according to any of the preceding claims, characterized in that a scheme of strands, which is used to build the prestressed concrete structure, is
devised according to the solved constrained non-linear optimization problem.
9. The method according to any of the preceding claims, characterized in that the inequality constraints are replaced by a system of equalities.
10. The method according to any of the preceding claims, characterized in that a system of non-linear equations is formed from the constrained non-linear optimization
problem by using a necessary Karush-Kuhn-Tucker condition, the system of non-linear
equations preferably being solved by using the Newton Method.
11. The method according to claim 10, characterized in that for solving the system of non-linear equations a start-vector for the prestressing
which refers to the curvature of bending moments resulting from dead-loads of the
structure is defined, and statically indeterminate parts of the prestressing are neglected
in a primary approach.
1. Verfahren zum Entwerfen und Bauen eines Spannbetonbauwerks, wobei das Entwerfen unter
Verwendung von Computern durchgeführt wird, wobei das Verfahren die folgenden Schritte
umfasst:
a) Modellieren der Aufgabe des Auffindens optimaler Parameter von Spannsträngen in
dem Spannbetonbauwerk in einem mathematischen Optimierungsproblem, und
b) Anwenden eines vorzugsweise gradientenbasierten numerischen Suchverfahrens zur
Lösung des mathematischen Optimierungsproblems, und
c) Bauen des Spannbetonbauwerks entsprechend den Ergebnissen aus Schritt b),
dadurch gekennzeichnet, dass in Schritt a) ein nichtlineares Optimierungsproblem mit Randbedingungen modelliert
wird, das eine zu optimierende Zielfunktion, durch Gleichheitsbeziehung bestimmte
Randbedingungen und durch Ungleichheitsbeziehung bestimmte Randbedingungen beinhaltet,
wobei sich die Zielfunktion vorzugsweise auf die Gesamtmenge des erforderlichen Strangmaterials,
insbesondere Stahl, in dem Spannbetonbauwerk bezieht, und
dass mechanische Gleichungen, die für die Zielfunktion und/oder für die durch Gleichheitsbeziehung
und/oder Ungleichheitsbeziehung bestimmten Randbedingungen verwendet werden, auf der
Grundlage von Prinzipien virtueller Arbeit angesetzt werden, wobei vorzugsweise das
Umwandeln statisch unbestimmter lasttragender Systeme in statisch bestimmte Systeme
über die Einführung virtueller Gelenke einbezogen wird.
2. Verfahren nach Anspruch 1, dadurch gekennzeichnet, dass mechanische Gleichungen, die für die Zielfunktion und/oder für die durch Gleichheitsbeziehung
und/oder Ungleichheitsbeziehung bestimmten Randbedingungen verwendet werden, auf der
Grundlage der Anwendung finiter Elemente als Grundelemente oder auf der Grundlage
von Prinzipien virtueller Kräfte und unter Verwendung von Spline-Funktionen angesetzt
werden.
3. Verfahren nach Anspruch 1 oder 2, dadurch gekennzeichnet, dass die durch Gleichheitsbeziehung bestimmten Randbedingungen und/oder durch Ungleichheitsbeziehung
bestimmten Randbedingungen relevante Bedingungen der Statik betreffen, bei denen wenigstens
einer der folgenden Strangparameter einbezogen wird: Vorspannkraft, Verlauf, Länge;
wobei die durch Gleichheitsbeziehung bestimmten Randbedingungen und/oder durch Ungleichheitsbeziehung
bestimmten Randbedingungen vorzugsweise ferner wenigstens eine der folgenden bestimmten
Bedingungen betreffen: abschnittsweise Errichtung des Bauwerks; zeitabhängiger Verlust
von Vorspannkräften aufgrund des Kriechens und Schwindens von Beton; Begrenzung von
Längs-, Druck- und Zugspannungen unter definierten Laststufen; reibungsbedingter Verlust
von Vorspannkraft längs der Stränge; Berücksichtigung ungekoppelter durchgehender
Stränge entlang breiter Bereiche des Bauwerks; Begrenzung der Längsspannungen aufgrund
von Biegemomenten und Axialkräften.
4. Verfahren nach einem der vorhergehenden Ansprüche, dadurch gekennzeichnet, dass bei Schritt a) eine virtuelle Unterteilung des Bauwerks in kleine aufeinanderfolgende
Abschnitte zur numerischen Beschreibung und Zuordnung mechanischer Eigenschaften des
Querschnitts für relevante Abschnitte des Bauwerks einbezogen wird, einschließlich
der Kraft relevanter Spannstränge und der Höhenlage der Stränge innerhalb des Querschnitts.
5. Verfahren nach einem der vorhergehenden Ansprüche, dadurch gekennzeichnet, dass das Ansetzen einer grundsätzlichen Optimierungsgleichung bei dem Optimierungsproblem
aus einem Verlauf von Biegemomenten und Axialkräften abgeleitet wird, die aus Last-
und Vorspannungseinwirkungen entstehen, wobei vorzugsweise wenigstens eines der folgenden
Elemente in der Grundgleichung enthalten ist: statisch bestimmte und statisch unbestimmte
Vorspannungsanteile; zeitabhängige Schwankung von Biegemomenten aufgrund des unterschiedlichen
Alters von Beton innerhalb aufeinanderfolgender Errichtungsabschnitte.
6. Verfahren nach Anspruch 5, dadurch gekennzeichnet, dass mittels einer Überlagerung statisch bestimmter und statisch unbestimmter Vorspannungsanteile
eine globale Gleichung für die gesamten vorspannungsbedingten Biegemomente je nach
den unbekannten Parametern der Stränge angesetzt wird, wobei die globale Gleichung
die Basis zur Formulierung der zu optimierenden Zielfunktion ist.
7. Verfahren nach einem der vorhergehenden Ansprüche, dadurch gekennzeichnet, dass es sich bei einer der Randbedingungen um die Bedingung handelt, dass die Kraft aller
seriellen Stränge nach rechts stets größer oder gleich dem relevanten, vorherigen
Strang nach links sein muss und umgekehrt, um die rechte Seite bzw. die linke Seite
für eine Zuweisung einer Vorspannankerhalterung festzulegen.
8. Verfahren nach einem der vorhergehenden Ansprüche, dadurch gekennzeichnet, dass gemäß dem gelösten nichtlinearen Optimierungsproblem mit Randbedingungen ein zum
Bauen des Spannbetonbauwerks verwendetes Strangschema ausgearbeitet wird.
9. Verfahren nach einem der vorhergehenden Ansprüche, dadurch gekennzeichnet, dass die durch Ungleichheitsbeziehung bestimmten Randbedingungen durch ein System von
Gleichheiten ersetzt werden.
10. Verfahren nach einem der vorhergehenden Ansprüche, dadurch gekennzeichnet, dass aus dem nichtlinearen Optimierungsproblem mit Randbedingungen unter Verwendung einer
notwendigen Karush-Kuhn-Tucker-Bedingung ein System aus nichtlinearen Gleichungen
gebildet wird, wobei das System aus nichtlinearen Gleichungen vorzugsweise durch Verwendung
des Newton-Verfahrens gelöst wird.
11. Verfahren nach Anspruch 10, dadurch gekennzeichnet, dass zur Lösung des Systems aus nichtlinearen Gleichungen ein Anfangsvektor für die Vorspannung
definiert wird, der sich auf die Krümmung von Biegemomenten bezieht, die sich aus
Eigenlasten des Bauwerks ergeben, und statisch unbestimmte Anteile der Vorspannung
in einem primären Ansatz vernachlässigt werden.
1. Procédé de conception et de construction d'une structure en béton précontraint, la
conception étant réalisée en utilisant des ordinateurs, le procédé comprenant les
étapes suivantes :
a) la modélisation du problème à trouver des paramètres optimaux de précontrainte
de fils dans la structure en béton précontraint dans un problème d'optimisation mathématique
; et
b) l'utilisation d'une méthode de recherche numérique de préférence basée sur un gradient
pour résoudre le problème d'optimisation mathématique ; et
c) la construction de la structure en béton précontraint conformément aux résultats
de l'étape b) ;
caractérisé en ce qu'à l'étape a), un problème d'optimisation non-linéaire sous contraintes est modelé,
incluant une fonction objectif à optimiser, des contraintes d'égalité et des contraintes
d'inégalité, la fonction objectif se rapportant de préférence à la quantité totale
de matière de fils nécessaire, en particulier de l'acier, dans la structure en béton
précontraint ; et
en ce que des équations mécaniques utilisées pour la fonction objectif et/ou pour les contraintes
d'égalité et/ou d'inégalité sont formulées sur la base de principes de travail virtuel,
impliquant de préférence la transformation de systèmes portant des charges statiquement
indéterminés en systèmes statiquement déterminés par l'introduction de joints virtuels.
2. Procédé selon la revendication 1, caractérisé en ce que des équations mécaniques utilisées pour la fonction objectif et/ou pour les contraintes
d'égalité et/ou d'inégalité sont formulées sur la base de l'utilisation d'éléments
finis comme éléments de base, ou sur la base de principes de forces virtuelles et
en utilisant des fonctions splines.
3. Procédé selon la revendication 1 ou 2, caractérisé en ce que les contraintes d'égalité et/ou les contraintes d'inégalité se rapportent à des conditions
pertinentes de statique incluant au moins l'un des paramètres de fil suivants : force
de précontrainte, parcours, longueur ; les contraintes d'égalité et/ou les contraintes
d'inégalité se rapportant de préférence en outre à au moins l'une des conditions particulières
suivantes : construction sectionnable de la structure ; perte de forces de précontrainte
en fonction du temps en raison d'un fluage et d'un retrait du béton ; limitation d'efforts
longitudinaux, de compression et de traction sous des niveaux de charge définis ;
perte de force de précontrainte le long des fils par friction ; prise en considération
de fils non-couplés continus le long de grandes zones de la structure ; limitation
des efforts longitudinaux en raison de couples de flexion et de forces axiales.
4. Procédé selon l'une des revendications précédentes, caractérisé en ce que l'étape a) comprend le partage virtuel de la structure en petites sections subséquentes
pour la description numérique et l'association de propriétés mécaniques de la section
transversale pour des sections pertinentes de la structure, y compris la force de
fils de précontrainte pertinents et l'élévation des fils à l'intérieur de la section
transversale.
5. Procédé selon l'une des revendications précédentes, caractérisé en ce que la formulation d'une équation d'optimisation de base dans le problème d'optimisation
est dérivé d'un développement de couples de flexion et de forces axiales résultant
des effets de charge et de précontrainte, au moins l'un des éléments suivants étant
de préférence compris dans l'équation de base : des parts de précontrainte statiquement
déterminées et statiquement indéterminées ; la variation de couples de flexion en
fonction du temps en raison de l'âge différent du béton dans des sections de construction
successives.
6. Procédé selon la revendication 5, caractérisé en ce que grâce à une superposition de parts de précontrainte statiquement déterminées et statiquement
indéterminées, une équation globale est formulée pour l'ensemble des couples de flexion
induits par une précontrainte en fonction des paramètres inconnus des fils, l'équation
globale étant la base pour la formulation de la fonction objectif à optimiser.
7. Procédé selon l'une des revendications précédentes, caractérisé en ce que l'une des contraintes est la condition selon laquelle la force de l'ensemble des
fils sériels à droite doit toujours être plus élevée ou égale au fil précédent pertinent
à gauche et vice versa, afin de déterminer le côté droit ou le côté gauche, respectivement,
pour l'attribution d'une fixation d'ancrage par précontrainte.
8. Procédé selon l'une des revendications précédentes, caractérisé en ce qu'un schéma de fils qui est utilisé pour construire la structure en béton précontraint
est établi selon le problème d'optimisation non-linéaire sous contraintes résolu.
9. Procédé selon l'une des revendications précédentes, caractérisé en ce que les contraintes d'inégalité sont remplacées par un système d'égalités.
10. Procédé selon l'une des revendications précédentes, caractérisé en ce qu'un système d'équations non-linéaires est réalisé à partir du problème d'optimisation
non-linéaire sous contraintes en utilisant une condition de Karush-Kuhn-Tucker nécessaire,
le système d'équations non-linéaires étant de préférence résolu en utilisant la méthode
de Newton.
11. Procédé selon la revendication 10, caractérisé en ce que pour résoudre le système d'équations non-linéaires, un vecteur initial pour la précontrainte
qui se rapporte à la courbure de couples de flexion résultant de poids morts de la
structure est défini, et en ce que des parts statiquement indéterminées de la précontrainte sont négligées dans une
approche primaire.