FIELD OF THE INVENTION
[0001] The present invention, in some of its embodiments, relates to apparatus and methods
of tomography in the field of nuclear medicine (sometimes referred to herein as "N-M
tomography"), and more particularly to N-M tomography systems as a whole, collimated
detector configurations for N-M tomography systems, and methods of using N-M tomography
systems and detectors. In some embodiments, the invention relates to modular N-M tomography
systems and to methods of upgrading existing N-M tomography systems. In some embodiments,
the invention relates to dual use SPECT-PET N-M tomography systems and detectors.
CROSS REFERENCE TO RELATED APPLICATIONS
BACKGROUND OF THE INVENTION
In General:
[0003] Systems and methods for medical imaging based on detection of particles emitted by
decay of radioactive tracer compounds injected into the bloodstream of a subject have
become important diagnostic and research tools, for example, in the fields of cardiology,
oncology, and neurology, as well in pre-clinical (e.g., small-animal) studies.
[0004] There are two major types of such imaging systems, single photon emission tomography
(SPECT) and positron emission tomography (PET). SPECT and PET systems both rely on
detection of gamma-ray photons resulting from decay of radio-isotopes, the concentration
of which in tissues is indicative of metabolic or other processes within the tissues.
For example, tumors are characterized by high metabolic activity and therefore high
uptake of the tracer compound, and correspondingly, higher gamma photon emission,
while normal tissue exhibits relatively lower metabolic activity and therefore lower
uptake of the tracer compound and lower photon emission.
[0005] Emission data is obtained at multiple positions around and along the region of interest
(ROI) to produce data representing a succession of 2D images or projections which
are then converted by use of suitable computerized image reconstruction algorithms
into 3D images.
[0006] Fig. 1A illustrates schematically major external features of an exemplary N-M tomography
system, generally designated at 10. The illustration is broadly representative of
the major external features of both conventional SPECT and PET systems, and of some
embodiments of the present invention.
[0007] System 10 is comprised of a gantry 12 mounted in a stationary frame 14, and a patient
carrier 16 including a patient bed arranged to move vertically within the gantry,
and axially through the gantry on a track 19. In some installations, patient carrier
16 is stationary, and gantry 12 is axially moveable along the patient carrier.
[0008] In conventional SPECT systems, gantry 12 is a ring-like structure arranged to rotate
in frame 14 around an axis that runs along the length of the patient carrier 16. (For
convenience, this will be referred to herein as the "system axis" or the "patient
axis".) The emission detectors in SPECT systems, or "cameras" as they are usually
referred to, are typically comprised of a small number, for example, one to four detector
units configured as flat plates, sometimes square and sometimes rectangular, extending
longitudinally in the direction of the system axis, i.e., into the plane of Fig. 1.
When two detector units are used, they are often positioned 90 degrees apart on the
gantry.
[0009] Relative axial movement between the gantry and the patient bed produces the succession
of 2D emission projections from which the 3D images are reconstructed. After reconstruction,
a "slice" corresponding to an axial position may be selected.
[0010] Fig. 1B shows another SPECT system configuration that is particularly useful for
cardiac studies of various kinds. The device, generally denoted at 20 employs an adjustable
chair 22 as the patient carrier. The detectors are located in a housing 24 moveably
attached to a main system housing 26.
[0011] The detector housing 24 is generally L-shaped, and the detectors shown by dash lines
28 extend transversely to the housing and generally along the patient axis in an arc
around the patient's torso.
[0012] A SPECT system configured as illustrated is available commercially at the time of
filing of this application from the applicant hereof under the name D-Spect®.
[0013] As noted above, the external features of conventional PET systems are similar to
those of conventional SPECT systems as illustrated in Fig. 1A. However, the detector
heads of a PET system are typically mounted on a non-rotating gantry ring surrounding
the patient bed. In typical commercial installations, the gantry ring is fully populated
by a large number of closely spaced detectors, each comprised of one or more individual
detector elements having small areas, to provide 360 degree coverage around an ROI.
PET detectors typically do not include collimators.
[0014] Other configurations have also been proposed.
U.S. patent 6,137,109 pertains to SPECT and positron coincidence detection (PET) systems having a small
number of detector heads, e.g., two or three, arranged in a polygonal configuration
around a gantry. Provision is made for the detector heads to be moved radially toward
the patient examination area and tangentially on the gantry to preset the bore size,
before an examination is performed.
[0015] Cherry, et al, Physics in Nuclear Medicine, Third Ed. p. 346, shows a PET system having a gantry ring partially populated by a small number of
detector heads arranged in a uniformly or non-uniformly spaced relationship on the
ring. In such constructions, to obtain data needed for full image reconstruction,
i.e., to provide full 360 degree coverage, the gantry must be rotated to a succession
of angular positions. However, a typical gantry installation including detector head
positioning mechanisms can weigh hundreds of kilograms, and repositioning the gantry
between images can require several minutes. This can be a significant factor in the
time required for a scan. Possibly due to the lower efficiency and prolonged acquisition
time, fixed-diameter rotating-only partly populated gantries have not been adopted
commercially.
[0016] A PET installation also generally includes a cyclotron (not shown) which is needed
for production of the isotopes used as tracers on site, or at a nearby facility. These
isotopes have very short half-lives, i.e. they decay quite rapidly, and often cannot
practically be used if produced off-site at a distant facility.
Some Differences Between PET and SPECT Systems and Methods:
[0017] SPECT and PET systems are structurally and functionally different in several important
respects, and have generally different advantages and limitations.
Basic Physics:
[0018] In PET imaging, as a radioactive isotope of the tracer undergoes decay, it emits
a positron, an antiparticle of the electron with opposite charge. The emitted positron
travels in tissue for a short distance (typically about 1 mm), losing energy as it
travels, to a point where it can interact with an electron. The interaction annihilates
both the electron and the positron, producing a pair of gamma ray photons having energies
of (typically) 511 KeV that move in approximately opposite directions. These high
energy photons are detected as a "paired event" (often referred to as "coincidence")
by an opposed pair of detectors. Signals from the detectors are collected and temporally
correlated to find such pairs and used to generate or reconstruct the 3D images.
[0019] For SPECT imaging, radioactive decay of the tracer isotope results in emission of
single gamma-ray photons without the intermediate step of positron-electron annihilation,
These photons typically have energies in the range of about 40-245 KeV, and are detected
as singular events.
Radiotracers:
[0020] Isotopes used in PET imaging typically have short half-lives (i.e., exhibit rapid
decay). Examples include carbon-11 (approximately 20 min half-life), nitrogen-13 (approximately
10 min), oxygen-15 (approximately 2 min), fluorine-18 (approximately 110 min), or
rubidum-82 (approximately 1.27 min). The most commonly used radiotracer in PET imaging
is fluorodeoxyglucose (also called FDG or fludeoxyglucose), an analogue of glucose
labeled with fluorine-18.
[0021] For SPECT imaging, the radioisotopes can be a simple soluble dissolved ion, such
as a radioisotope of gallium. Alternatively, and most commonly, a marker radioisotope
is attached to another compound which is of interest for its ability to bind in a
medically interesting way to tissue under investigation.
[0022] Typical tracers used in SPECT imaging include technetium-99ml (the most commonly
used), iodine-123, or iodine 131, or indium-111. These isotopes are heavier than those
used in PET and exhibit half-lives measured in hours or even days.
[0023] As noted above, cells exhibiting high metabolic activity, for example, cancer cells,
typically strongly absorb the radioisotope. In both PET and SPECT imaging, the intensity
of the gamma-ray emission provides a measure of concentration in the tissue.
Detectors:
[0024] Since both PET and SPECT imaging involve detection of gamma photons, both can utilize
detectors that function by the same basic operating principle. The original SPECT
detector was the so-called "Anger Camera" (named after its inventor) developed in
the 1950's, and while the technology has evolved over time, detectors employing the
operating principle of the Anger camera are still used in the majority of commercial
SPECT installations.
[0025] Fig. 2A shows schematically the construction of an Anger Camera as configured for
SPECT imaging, generally designated at 30. Camera 30 is comprised of a detector element
32, usually formed of a thallium-doped sodium iodide (NaI:Tl) crystal optically coupled
to an array of photomultipliers (PMT) 34 positioned behind detector element 32. Typically,
there are 30-60 or more PMTs depending on the sizes of the detector and the PMTs,
arranged in a square or rectangular array.
[0026] NaI:Tl crystal 32 functions as a scintillator to convert gamma-ray emissions from
a radioactive tracer that has been injected into the blood stream of a subject 36
to visible or near ultraviolet light pulses. PMTs 34 convert the light pulses produced
by crystal 32 into electrical output signals which are provided to a computer 38 programmed
according to a desired algorithm to reconstruct a succession of 2D slice images into
an ultimate 3D image. The 3D image is then stored electronically and/or provided to
a visual display unit 40.
[0027] Many or all of PMTs 34 simultaneously detect the (presumed) same flash of light to
varying degrees, at intensities generally depending on their position relative to
the actual emission event. In conventional practice, spatial information about the
locations of the gamma photon emissions is obtained by placing a collimator 42 in
front of the detection crystal/PMT array. The collimator consists of a thick sheet
of lead, typically 1-3 inches thick, with thousands of adjacent holes through it which
limits the direction from which the photons can reach crystal 32. The holes are sometimes
defined by septa, which block radiation at other directions. In some cases, the collimator
is a machined metal plate.
[0028] SPECT cameras and especially Anger-type SPECT cameras, use collimators to ensure
that photons striking the detectors do so at a relatively narrow range of angles.
However, the collimator absorbs a substantial percentage of the incident photons.
This limits the sensitivity of the camera system, thereby increasing the time required
to obtain sufficient data for a good image. Other methods of image localization, for
example, different collimator configurations, and other scintillation detector materials
have been proposed but the classic Anger camera still dominates in commercial use.
Compton cameras do not use a collimator for detecting the direction, instead scattering
of the gamma ray from one set of detectors is detected by a second set of detectors
and the path of the gamma ray reconstructed therefrom.
[0030] Fig. 2B is a block diagram that illustrates schematically major electronic features
of an exemplary conventional PET system, generally designated at 50. Illustrative
system 50 includes a detector signal input unit 52 which receives and pre-processes
the signal outputs of the detector heads received over a set of signal paths 54, As
will be understood, for PET scans, a main function of input unit 54 is to perform
coincidence detection of paired signals. As will also be understood, for SPECT imaging,
the detector head outputs are processed without the need for coincidence detection.
[0031] Output signals from Input Unit 22 are provided to an Image Reconstruction Unit 56,
typically a suitably programmed microprocessor-based system, by which the 3D images
of the ROIs are created from the detected photon patterns.
[0032] The reconstructed images are provided to a display sub-system 58, typically including
a user interface, e.g., a keyboard and mouse or other input device (not shown) and
a display unit 58.
[0033] The operation of the system of Fig. 2B is controlled by a system controller 30, which
is typically comprised of a suitably programmed microprocessor, possibly the same
one that performs image reconstruction.
[0034] As mentioned above, PET imaging relies on coincidence detection of two oppositely
traveling photons, and therefore different detector configurations are conventionally
used for PET and SPECT imaging. One notable difference is that collimators are not
needed for PET imaging since the required spatial resolution results from the coincidence
detection process. The absence of a collimator also results in improved sensitivity.
[0035] Correspondingly, different image reconstruction algorithms are used for PET and SPECT
imaging. One PET image reconstruction algorithm known to those skilled in the art
include ML-EM algorithm. Among SPECT image reconstruction algorithms known to those
skilled in the art are iterative reconstruction and back projection.
[0038] The contents of each of these documents are incorporated herein by reference as if
fully set forth.
[0039] Both conventional PET and SPECT systems are widely used in the fields of oncology
and neurology, while SPECT systems are commonly used in cardiology, bone scan imagining,
and pre-clinical studies. Another factor relevant to choice of one or the other modality
is availability of the isotopes and tracers. Tc-99m is very commonly available for
SPECT while F-18 is commonly available for PET.
[0040] Each modality is commonly accepted to have certain advantages and limitations. For
example, PET systems have historically provided better spatial resolution and faster
examination, but are generally much more expensive than SPECT systems, mainly due
to the large number of detector elements needed to fully populate the gantry, and
the complex coincidence circuitry, but also partially due to the fact that the cyclotron
itself is costly and requires high-level technical support.
[0041] SPECT systems yield good results for many general purpose applications, including
in the fields of cardiology and small-animal pre-clinical imaging. For example, because
the gantry rotates during a scan, obtaining full 180 degree coverage may be easier.
However, despite technological advances, conventional SPECT systems still provide
lower resolution images than PET systems, and consequently, the SPECT images are generally
less detailed than PET images due at least in part to scatter and attenuation of the
lower energy of the photons. A SPECT scan also typically takes longer than a PET scan,
and requires a patient not to move for a longer time, or even to be immobilized and
limits the ability to visualize rapid functional changes. Also, the PET tracers' high
energy of the photons results in spatial resolution that is less dependent on distance
to the ROI.
[0042] Further, since SPECT detectors generally require collimators, there is an inherent
tradeoff between resolution and sensitivity.
[0043] Another limitation of conventional N-M tomography systems, especially PET systems,
is that reducing the number of detector heads to avoid the high cost of fully populating
the gantry ring as in
U.S. patent 6,137,109 and in
Cherry et al. mentioned above sometimes results in reduced spatial resolution and/or longer scan
times for scans of small ROIs in systems having a bore size large enough to accommodate
obese patients. This limitation is often addressed by the purchase of separate systems
for examining patients of large girth and for examining small ROIs.
[0044] Yet a further issue concerns the high initial cost of an N-M tomography system. Often,
a customer has a limited budget or limited needs which results in purchase of a system
having limited capability. For example, a facility faced with budgetary limitations
might choose to invest initially in a SPECT or a PET system, but not both, depending
on patient volumes and a decision to focus on cardiology or oncology and/or neurology.
However, as financial resources or needs change, an entirely new system may need to
be purchased. The same thing may happen when technical advances dictate a need for
improved capabilities.
[0045] Commonly owned technology that may be generally relevant to SPECT imaging, protocols
and doses, reconstruction and multi-dimensional imaging and image processing and is
optionally used together with some embodiments of the invention may be found in one
or more of the following International (PCT) or U.S. published applications or issued
U.S. patents:
PCT Published Applications:
Published U.S. Applications:
U.S. Patents:
[0048] 6,173,201;
6,368,331;
6,567,687 7,652,259;
7,705,316;
7,705,316;
7,826,889;
7,872,235;
7,968,851;
7,970,455;
8,000,773;
8,036,731;
8,094,894;
8,111,886;
8,280,124;
8,338,788
SUMMARY OF THE INVENTION
[0049] According to an aspect of some embodiments of the invention, there is provided an
N-M tomography system having a support for a subject of an examination procedure,
a plurality of detector heads, a carrier for the detector heads, and a detector positioning
arrangement operable to position the detector heads including rotation thereof to
adjacent or radially or circumferentially or axially overlapping positions before
and/or during performance of a scan without interference or collision between adjacent
detector heads to establish a variable bore size and configuration for the examination.
[0050] According to some embodiments, the detector heads include detectors which do not
move relative to said heads during acquisition.
[0051] According to some embodiments, the system includes a processor which uses data collected
by all of said detector heads, for image reconstruction.
[0052] According to some embodiments, the detector positioning arrangement is operable to
move at least some of the detectors toward and away from the subject carrier to determine
the detector bore size.
[0053] According to some embodiments, the movement of at least two detector heads is off-center
of a central axis of said bore and not towards any common parallel axis of said bore.
[0054] According to some embodiments, the movement of at least one detector heads is not
along a straight line.
[0055] According to some embodiments, the detector positioning arrangement is operable to
move at least some of the detectors to a desired rotational orientation relative to
respective longitudinal axes thereof.
[0056] According to some embodiments, the detector positioning arrangement is operable to
tilt at least some of the detector heads to a desired orientation relative to respective
longitudinal axes thereof.
[0057] According to some embodiments, the detector positioning arrangement is operable to
adjust the bore size preliminary to a scan, or in steps at selected axial and/or circumferential
positions during a scan, or continuously as the scan proceeds.
[0058] According to some embodiments, the detector positioning arrangement is comprised
of separate mechanisms to extend and retract and to angularly orient the detector
heads.
[0059] According to some embodiments, detector-bearing parts of at least some detector heads
are tilted by rotation around an axis parallel to a system axis.
[0060] According to some embodiments, one of the separate mechanisms for extending and retracting,
and angularly orienting the detector heads is associated with each detector head.
[0061] According to some embodiments, the detectors heads are all PET detector heads or
all SPECT detector heads, or a combination of SPECT and PET detector heads or dual
purpose detector heads.
[0062] According to some embodiments, the system includes a drive mechanism to rotate the
detector heads around the subject carrier to provide 360 degree coverage around the
patient carrier with substantially no gaps for a selected bore size.
[0063] According to some embodiments, the detector heads are positioned non-uniformly around
the detector carrier.
[0064] According to some embodiments, the detector positioning arrangement is operable to
orient the detector heads angularly to prevent interference between adjacent detector
heads when the detector heads are extended to a desired position.
[0065] According to some embodiments, the detector carrier is comprised of two or more rings
spaced apart from each other by an air gap along a longitudinal axis of the subject
carrier with detector heads mounted on each ring.
[0066] According to some embodiments, the detector carrier is a single ring with detector
heads axially spaced on one side of the single ring, or on opposite sides of the single
ring.
[0067] According to some embodiments, a plurality of pairs of PET detector heads are spaced
circumferentially around the detector head carrier, such that adjacent pairs of PET
detector heads are in a spaced relationship on the longitudinal axis of the subject
carrier.
[0068] According to some embodiments, at least some of the detector heads are circumferentially
or laterally moveable along a path near the periphery of the detector carrier.
[0069] According to some embodiments, the detector carrier is configured to accommodate
a variable number of detector heads.
[0070] According to some embodiments, the system includes a controller which controls the
detector positioning arrangement to provide movements of the detectors.
[0071] According to some embodiments, each detector head is associated with its own detector
positioning arrangement.
[0072] According to an aspect of some embodiments of the invention, there is provided an
N-M tomography system having a plurality of detector heads mounted on a detector carrier
that is configured to be populated by a variable number of detector heads and a data
processing unit responsive to signal outputs from the population of detector heads
to reconstruct a tomographic image from the signals.
[0073] According to some embodiments, the detector carrier of an existing system is configured
to receive additional detector heads or replacement detector heads having different
operational characteristics as part of a system upgrade.
[0074] According to some embodiments, the detector carrier includes mounting receptacles
to which the additional or replacement detector heads can be operatively attached.
[0075] According to some embodiments, the detector heads include signaling mechanisms that
indicate the presence of a detector head installed at a particular position on the
carrier, and/or the functional characteristics of the installed detector heads.
[0076] According to some embodiments, the data processing unit is responsive to the signaling
mechanisms.
[0077] According to an aspect of some embodiments of the invention, there is provided an
N-M tomography system having a plurality of detector heads mounted on a detector carrier,
wherein the detector carrier is configured to be populated by detector heads of variable
type and/or quality; and a data processing unit responsive to signal outputs from
the population of detector heads to reconstruct a tomographic image from the signals.
[0078] According to an aspect of some embodiments of the invention, there is provided a
method of using an N-M tomography system that includes a support for a subject of
a tomography procedure and a detector arrangement comprised of plurality of detector
heads that are adjustably positionable on a detector carrier, in which the method
involves selecting a bore geometry for the detector array according to a particular
region of interest of a subject of a procedure by extending and angularly orienting
the detector heads such that adjacent detector heads do not interfere with each other
and, scanning the region of interest at successive axial positions relative to a patient
carrier.
[0079] According to some embodiments, the bore size is selected by one or more of: extending
at least some of the detectors toward the subject carrier, rotating at least some
of the detectors relative to respective longitudinal axes thereof, tilting at least
some of the detectors to a desired off-axis orientation relative to respective longitudinal
axes thereof, and moving at least some of the detector heads circumferentially or
laterally relative to the periphery of the detector carrier.
[0080] According to some embodiments, the selected bore geometry is varied as a scan proceeds.
[0081] According to some embodiments, the bore geometry is varied for at least some axial
positions.
[0082] According to some embodiments, the bore geometry is varied continuously as the scan
proceeds at a particular axial position.
[0083] According to some embodiments, the detector carrier rotates as the scan proceeds
at a particular axial position.
[0084] According to an aspect of some embodiments of the invention, there is provided a
method of upgrading an existing N-M tomography system that includes a support for
a subject of a tomography procedure, a detector arrangement comprised of a plurality
of SPECT detector heads and/or PET detector heads, in which one or more additional
detector heads of a desired type are installed in receptacles at pre-existing coupling
positions on the detector carrier.
[0085] According to some embodiments, the method further includes installing adjacent PET
detector pairs in receptacles at different axial spacing relative to a subject carrier.
[0086] According to some embodiments, the method further includes positioning at least one
additional detector carrier in spaced relationship with an existing detector carrier
along a longitudinal axis of the subject carrier.
[0087] According to some embodiments, an existing detector head is replaced in the field,
with a detector head of different quality or type.
[0088] According to an aspect of some embodiments of the invention, there is provided a
detector unit for a Nuclear Medicine (NM) imaging system having a detector element
responsive to gamma ray photons to provide an electrical output signal and a collimator
formed of first and second sets of septa extending in two directions that intersect
to define an array of collimator cells, in which the septa are moveable to change
the geometry of the collimator to increase and decrease the spatial resolution of
the detector unit.
[0089] According to some embodiments, the septa of the first set are equally spaced from
each other and the septa of the second set are equally spaced from each other.
[0090] According to some embodiments, the spacing between the septa of the first set is
equal to the spacing between the septa of the second set.
[0091] According to some embodiments, the spacing between the septa of the first set is
unequal to the spacing between the septa of the second set.
[0092] According to some embodiments, all the septa of the first and second sets are respectively
parallel to each other.
[0093] According to some embodiments, all the septa of at least one of the sets are not
parallel to each other.
[0094] According to some embodiments, the septa of at least one of the sets are moveable
to increase the length thereof relative to a surface of the detector element.
[0095] According to some embodiments, the septa are moveable to increase or decrease the
septa spacing in one and/or both directions relative to a surface of the detector
module.
[0096] According to some embodiments, the collimator is formed of a plurality of parts spaced
from each other in a direction perpendicular to a surface of the detector element,
each part being formed of first and second sets of intersecting septa, and the spacing
is increased or decreased by moving one or both parts parallel to a surface of the
detector element.
[0097] According to some embodiments, the collimator is formed of two or three spaced parts.
[0098] According to some embodiments, the collimator is formed of three parts perpendicularly
spaced relative to the detector element, wherein the septa of the intermediate part
are tiltable.
[0099] According to some embodiments, at least some the septa in one or both sets are tiltable
relative to a surface of the detector element.
[0100] According to some embodiments, one end of the collimator cells includes a shutter
to adjust the effective size of the area exposed to incoming photons.
[0101] According to some embodiments, the shutter is slidable or tiltable or in the form
of an iris.
[0102] According to some embodiments, the septa are comprised of plates having spaced slots
therein, wherein the spacing of the slots in the septa of the first set match the
spacing of the septa of the second set, and the spacing of the slots in the septa
of the second set match the spacing of the septa of the first set.
[0103] According to some embodiments, the septa of the first set are oriented orthogonally
to the septa of the second set.
[0104] According to some embodiments, the spacing of the septa of the first and second set
is non-uniform whereby collimator cells at different locations in the collimator are
of different sizes.
[0105] According to some embodiments, the collimator cells are square.
[0106] According to some embodiments, the collimator cells are rectangular.
[0107] According to some embodiments, the septa in the first and/or second sets are of non-uniform
thickness.
[0108] According to some embodiments, the septa of the first and second sets are of different
lengths.
[0109] According to some embodiments, the septa of the first and/or second sets are respectively
of varying lengths.
[0110] According to some embodiments, the detector element is pixilated.
[0111] According to some embodiments, the septa are positioned at the borders of each pixel,
whereby the collimator cells are aligned with the detector pixels with one pixel per
collimator cell.
[0112] According to some embodiments, the spacing of the septa of the first and/or second
sets is greater than the pixel pitch.
[0113] According to some embodiments, the spacing of the septa of the first and/or second
sets is smaller than the pixel pitch.
[0114] According to some embodiments, the septa of the first and/or second sets are respectively
not parallel to each other.
[0115] According to some embodiments, the collimator cells are of different sizes and are
also of different in size from the size of the detector element pixels.
[0116] According to some embodiments, the pixels are arranged in a square matrix or a rectangular
matrix.
[0117] According to some embodiments, the detector element is a single crystal scintillator
and is optically coupled to an array of PMTs.
[0118] According to some embodiments, the detector element is an array of direct conversion
semiconductor detectors.
[0119] According to some embodiments, the detector element is configured as an array of
SiPMs.
[0120] According to some embodiments, the detector elements are formed of CZT or LYSO.
[0121] According to some embodiments, the septa are formed of tungsten.
[0122] According to some embodiments, the septa are configured to block less than 50% of
511Kev radiation passing through at a thickness dimension thereof.
[0123] According to an aspect of some embodiments of the invention, there is provided a
collimator for attachment to a Nuclear Medicine (NM) imaging system detector element
responsive to gamma ray photons to provide an electrical output signal, formed by
first and second sets of septa extending in two directions that intersect to define
an array of collimator cells, in which the septa are moveable to change the geometry
of the collimator to increase and decrease the spatial resolution of the detector
unit.
[0124] According to an aspect of some embodiments of the invention, there is provided a
collimator for attachment to a Nuclear Medicine (NM) imaging system detector element
responsive to gamma ray photons to generate an electrical output signal, having a
body defining first and second sets of septa extending in two directions, in which
a thickness and/or height of the septa in one direction is different from a thickness
and/or height in another direction.
[0125] According to an aspect of some embodiments of the invention, there is provided a
detector unit for a Nuclear Medicine (NM) imaging system having a detector element
responsive to gamma ray photons having energy in the range of about 40 KeV to 511
KeV, and a collimator configured to permit use of the detector for both PET and SPECT
imaging.
[0126] According to some embodiments, the detector element is a scintillator and the detector
unit further includes an array of PMTs optically coupled to the detector element to
provide electrical output signals.
[0127] According to some embodiments, the detector element is a direct conversion element
operative to generate an electrical output signal in response to impingement of a
gamma ray photon.
[0128] According to some embodiments, an electronic signal processor is selectably operable
to reconstruct a PET image or a SPECT image or operable to simultaneously reconstruct
PET and SPECT images.
[0129] According to some embodiments, the circuitry is configured to selectively process
a signal from the detector as a single photon event or as a coincidence event.
[0130] According to some embodiments, the geometry of the collimator is adjustable to provide
high and low spatial resolution.
[0131] According to some embodiments, the collimator septa are formed of a material that
absorbs PET energy photons with an efficiency of about 50 percent or less of its efficiency
of absorbing SPECT photons.
[0132] According to some embodiments, the collimator septa are formed of tungsten.
[0133] According to an aspect of some embodiments of the invention, there is provided a
method of imaging using an N-M tomography system, in which both PET and SPECT data
from a target area are simultaneously collected using a single set of detectors.
[0134] According to some embodiments, the geometry and/or position of detector heads forming
a detector array is adjusted relative to the target area.
[0135] According to some embodiments, multiple radiopharmaceutical tracers providing photon
emissions having different energies are employed.
[0136] According to some embodiments, the detectors are comprised of radiation detector
elements and collimators that are adjustable to provide different degrees of spatial
resolution.
[0137] According to an aspect of some embodiments of the invention, there is provided a
method of imaging using an N-M tomography system having a collimated detector unit,
in which the configuration of the collimator septa are adjusted to provided a desired
degree of spatial resolution; and SPECT imaging is performed using an algorithm that
assumes a collection angle and a detection probability map according the adjustment
of the collimator configuration, or PET imaging is performed taking account of a detection
probability resulting from the adjusted variable septa geometry such that the probability
is factored into the image reconstruction.
[0138] According to an aspect of some embodiments of the invention, there is provided a
method of imaging using an N-M tomography system having a plurality of rotating detector
units, in which the rotation of the detector units is adjusted to provide a desired
degree of spatial resolution and/or bore size and SPECT imaging is performed using
an algorithm that assumes a collection angle and a detection probability map according
the adjustment, or PET imaging is performed taking account of a detection probability
resulting from the adjustment such that the probability is factored into the image
reconstruction.
[0139] According to some embodiments, there is provided an N-M tomography system in which
the detector heads are formed by a detector element responsive to gamma ray photons
to provide an electrical output signal and a collimator having first and second sets
of septa extending in two directions that intersect to define an array of collimator
cells that septa are moveable to change the geometry of the collimator to increase
and decrease the spatial resolution of the detector unit.
[0140] According to some embodiments, the detector heads are formed of a detector element
response to gamma ray photons having energy in the range of about 40 KeV to 511 KeV
and a collimator configured to permit use of the detector for both PET and SPECT imaging.
[0141] Implementation of the method and/or system of embodiments of the invention can involve
performing or completing selected tasks manually, automatically, or a combination
thereof. Moreover, according to actual instrumentation and equipment of embodiments
of the method and/or system of the invention, several selected tasks could be implemented
by hardware, by software or by firmware or by a combination thereof using an operating
system.
[0142] For example, hardware for performing selected tasks according to embodiments of the
invention could be implemented as a chip or a circuit. As software, selected tasks
according to embodiments of the invention could be implemented as a plurality of software
instructions being executed by a computer using any suitable operating system. In
an exemplary embodiment of the invention, one or more tasks according to exemplary
embodiments of method and/or system as described herein are performed by a data processor,
such as a computing platform for executing a plurality of instructions. Optionally,
the data processor includes a volatile memory for storing instructions and/or data
and/or a non-volatile storage, for example, a magnetic hard-disk and/or removable
media, for storing instructions and/or data. Optionally, a network connection is provided
as well. A display and/or a user input device such as a keyboard or mouse are optionally
provided as well.
DESCRIPTION OF THE DRAWINGS
[0143] Some embodiments of the invention are herein described, by way of example only, with
reference to the accompanying drawings. With specific reference now to the drawings
in detail, it is stressed that the particulars shown are by way of example and for
purposes of illustrative discussion of embodiments of the invention. In this regard,
the description taken with the drawings makes apparent to those skilled in the art
how embodiments of the invention may be practiced.
[0144] In the drawings:
FIG. 1A is an exemplary schematic illustration of major external features of a N-M
tomography system;
FIG. 1B is an exemplary schematic illustration of major external features of another
commercially available SPECT system configuration;
FIG. 2A is an exemplary schematic illustration of the layout of a detector unit, for
example, an Anger camera for a SPECT system;
FIG. 2B is an exemplary schematic illustration of an electronic subsystem for z PET
system;
FIGs. 3A-3D illustrate the concept of modularity of a detector head array in an N-M
tomography system in the context of an exemplary SPECT or PET system according to
some embodiments of the invention;
FIGs. 4A and 4B illustrate upgrading an as-built single-mode PET or SPECT system into
a dual-mode SPECT+PET system according to some embodiments of the invention;
FIG. 5 is an enlarged version of FIG. 4B according to some embodiments of the invention;
FIGs. 6A and 6B illustrate the concept of variable bore size according to some embodiments
of the invention;
FIGs. 6C and 6D illustrate advantages of variable bore size as implemented according
to some embodiments of the invention;
FIG. 6E is a side perspective view that illustrates schematically a linear motion
mechanism according to some embodiments of the invention;
FIG. 6F is a side elevation of FIG. 6E;
FIG. 6G illustrates an exemplary mechanism for extending and retracting a detector
head according to some embodiments of the invention;
FIGs. 7A and 7B illustrate a way to achieve variable bore size shown by way of example
for a system operating in a PET mode according to some embodiments of the invention;
FIGs. 8A-8D illustrate another way to achieve variable bore size shown by way of example
for a system operating in a PET mode according to some embodiments of the invention;
FIGs. 9A-9C illustrate yet another way to achieve variable bore size shown by way
of example for a system operating in a PET mode according to some embodiments of the
invention;
FIGs. 10A and 10B illustrate still another way to achieve variable bore size shown
by way of example for a system operating in a PET mode according to some embodiments
of the invention;
FIGs. 11A-11D illustrate still another way to achieve variable bore size according
to some embodiments of the invention;
FIG. 12A illustrates an exemplary embodiment in which detector heads are translatable
linearly on a gantry;
FIG. 12B illustrates an exemplary embodiment in which detector heads are translatable
circumferentially on a gantry;
FIG. 13 illustrates benefits of continuous rotation of a detector carrier according
to some embodiments of the invention;
FIG. 14 illustrates an arrangement for dynamically varying the bore size during a
scan;
FIG. 15 is a flow diagram illustrating use of an N-M tomography system according to
other embodiments of the invention;
FIG. 16 is a flow diagram illustrating use of an N-M tomography system according to
some other embodiments of the invention;
FIG. 17 illustrates the construction and assembly of a basic collimator;
FIGs. 18A-18C illustrate some exemplary (but non-limiting) ways to vary the resolution
of collimators according to some embodiments of the invention by lengthening or shortening
the collimator septa;
FIGs. 19A and 19B illustrate exemplary (but non-limiting) ways to vary the resolution
of collimators according to some embodiments of the invention by sliding the septa
laterally;
FIGs. 20A and 20B illustrate exemplary (but non-limiting) ways to vary the resolution
of collimators according to some embodiments of the invention by tilting and/or shifting
the septa;
FIGs. 21A-21D illustrate resolution adjustment using an arrangement of layered or
vertically tandem collimator sub-units or parts, in accordance with some embodiments
of the invention;
FIGs. 22A-22D illustrate exemplary (but non-limiting) ways to vary the resolution
of collimators according to some embodiments of the invention using adjustable shutters;
and
FIG. 23A-23C illustrates representative (qualitative) performance of collimator constructions
and configurations according to some embodiments of the invention.
[0145] In conjunction with the following detailed description of various aspects of some
embodiments of the invention, it is to be understood that the invention is not necessarily
limited in its application to the details of construction and the arrangement of the
components and/or methods set forth in the following description and/or illustrated
in the drawings and/or the Examples. The invention is capable of other embodiments
or of being practiced or carried out in various ways.
DETAILED DESCRIPTION OF THE INVENTION
Introductory Overview:
[0146] The present invention, in some of its embodiments, relates to apparatus and methods
of tomography in the field of nuclear medicine (sometimes referred to herein as "N-M
tomography"), and more particularly to N-M tomography systems as a whole, to detector
configurations for N-M tomography systems, and methods of using and/or upgrading such
systems and detector arrangements. In some embodiments, the invention also relates
to methods of upgrading existing N-M tomography systems and detectors.
[0147] In an exemplary embodiment of the invention, there is provided a single system which
is easily (e.g., field) upgradable and also capable of dual-mode functionality, e.g.,
having the capability of selectably operating in either SPECT or PET mode (or both)
without materially sacrificing performance in either mode. In an exemplary embodiment
of the invention, there is a provided a single detector that can be selectably operable
in either a SPECT a PET mode with good resolution and sensitivity.
[0148] In an exemplary embodiment of the invention, there is a provided a system to be capable
of operating simultaneously in both a SPECT and a PET mode.
[0149] Some embodiments of the present invention are directed to the issues discussed above,
and/or to other aspects of N-M tomography technology.
[0150] Aspects of some embodiments of the invention are equally applicable to single function
SPECT and PET systems, and to dual function SPECT and PET systems, unless otherwise
indicated.
[0151] An aspect of some embodiments of N-M tomography systems according to the present
invention resides in detector systems comprised of multiple detector heads (for example,
3-18 heads), each head including multiple individual detector elements (for example,
4-10 or more individual detector elements). The detector units are arranged to form
a bore defining a space within which the patient as a whole or a part of the patient's
body, i.e., an ROI, is examined.
[0152] In some of such embodiments, the detector heads are moveable (e.g., during imaging
and/or as a set up for an imaging session) relative to the patient carrier and/or
to each other and/or to the gantry in various ways allowing adjustment of the size
and/or shape of the bore according to the particular ROI without obstruction or collision
of adjacent detector heads. In some embodiments, the systems are operable to adjust
the size and shape of the bore during a scan.
[0153] Optionally, rapid reconfiguration (e.g., faster than 2 hours, 1 hour, 30 minutes,
10 minutes, 5 minutes per detector head) of the detector heads from one position and/or
orientation to another for step and shoot operation is facilitated by a light-weight
design of the detector heads and/or by counterbalancing.
[0154] Optionally, in some embodiments of the invention, the various degrees of freedom
can also be implemented in a horizontal system, in which the patient stands or sits,
e.g., such that system axis (as well as the main patient axis) is vertical or at an
angle to both vertical and perpendicular, and the gantry is relatively horizontal
(or at an angle, such as between 20 and 80 degrees to the horizontal.
[0155] In some embodiments of the invention, the system provides single-mode functionality,
in which case, the detector heads are comprised of only SPECT detectors, or only PET
detectors. In some embodiments, the system provides dual-mode functionality, in which
case, the detector system includes separate detector heads carrying PET and SPECT
detectors, or detector heads that include both PET and SPECT detectors.
[0156] Optionally, the detector heads are constructed of detectors that can selectably operate
as either PET or SPECT detectors or both SPECT and PET detectors simultaneously. Optionally,
such detector heads may include the detector elements, collimators, and/or circuitry
that can operate, for example, in single photon detection mode and in coincidence
mode. Optionally, timing circuitry is provided in a detector head and coincidence
circuitry in a more central location such as a CPU. Alternatively, coincidence circuitry
as well is provided in association with a detector head (e.g., module, possibly a
head on an arm), optionally the head receiving data on other detections from another
head, for example, via a system bus interconnecting heads.
[0157] In some embodiments, PET detector heads are T-shaped, or L-shaped (e.g., with wider
part facing the bore), and SPECT detector heads are rod or I-shaped. The detector
head include elongated stems that serve as the axis for extension/retraction and the
detector elements arrayed on the ends of the stems in various polygonal configurations
including square or rectangular or triangular, or in circular or arc-shaped configurations,
or combination thereof. Optionally, in rest positions, the detector elements extend
longitudinally in the direction of the system axis.
[0158] Optionally, the detector heads are comprised of a single detector element or a plurality
of detector elements forming a pixilated detector.
[0159] For convenience, the terms "detector arrangement" or "detector system" or "detector
unit" will sometimes be used in reference to multiple and single-detector heads, and
to heads that carry SPECT and/or PET detectors, and without distinction as to detector
head shape.
[0160] An aspect of some embodiments of N-M tomography systems according to the present
invention resides in variable-geometry detector systems in which the detector heads
are moveable relative to each other and to the gantry in various ways to improve sensitivity
and spatial resolution for imaging different ROIs.
[0161] An aspect of some embodiments resides in variable-geometry detector systems in which
the detector units are non-uniformly arranged on the gantry with (possibly) large
gaps between them wherein the adjustability of the detector heads still provides full
360 degree detector coverage possibly without loss (or with improvement) of sensitivity
and/or spatial resolution for differently sized and shaped ROIs and/or at different
positions along the body of a subject under examination. In some embodiments of the
present invention, such configurations possibly reduce the overall number of detectors
needed for a given level of spatial resolution and sensitivity, and thus reduce the
overall system cost.
[0162] The variable geometry features may allow trading off cost and imaging efficiency.
The detectors represent a major component in the cost of a system, so being able to
vary the bore size and shape according to the size of the patient and/or the location
and/or the size of the ROI, may allow generating good images with a smaller number
of detectors than would be needed in conventional fixed-geometry systems. However,
for a larger ROI or a larger patient, longer imaging times may result from reducing
the number of detectors. However, this may allow a majority of studies to be performed
fast and/or at a lower cost.
[0163] In some embodiments, the detector heads are extended and retracted by a linear extension
and retraction mechanism. Optionally, for operation in a PET mode, the detector heads
are extended individually or in opposed pairs. Optionally, the opposed pairs are diametrically
opposed.
[0164] Optionally, multiple detector heads are mounted on and/or moved together along a
single arm for in-out and/or lateral motion.
[0165] Optionally, at least some of the detector heads are not moved and data for imaging
is optionally collected from both moved and unmoved detector heads.
[0166] Optionally, a detector head includes electronic circuitry that supports more than
one set of separate detector elements. For example, one detector head may include
circuitry to support processing of signals from two detector heads which are connected
by a data cable. In another example, a detector head is set up to support multiple
types of detectors and/or collimators, which may be selectively mounted thereon. Optionally,
an RFID code or other machine readable indicator on the detector and/or collimator
serve to indicate to the processing circuitry (e.g., in arm or in main machine) which
type of detection is available and/or to guide data acquisition, acquisition planning
and/or reconstruction, according to the ability of the detector. Optionally, the indicator,
or a different storage location includes a table or a set of parameters matching the
type of detector and parameters or software for using the detector.
[0167] If the size and/or shape of the individual detector heads; (particularly but not
exclusively in the case of PET detector heads) do not permit sufficient extension
to reduce the bore size to a desired degree without collision or interference between
adjacent detector heads, several options are available in accordance with some embodiments
of the invention. In some embodiments, only some of the detector heads are extended.
For example, every other detector head (i.e., one-half the total number of detector
heads) are extended, and the others remain un-extended. Optionally, the un-extended
detector heads are not used during the scan. Optionally, the un-extended detector
heads and the extended detector heads are used during the scan.
[0168] Alternatively, the angular orientation of at least some or all of the detector heads
may be varied relative to the axes of extension of the respective detectors to increase
the amount that the detectors can be extended without collision. This can be advantageous
since allowing a greater range of bore size adjustability can, potentially, better
accommodate differently sized and shaped ROIs and ROIs at different locations along
the subject's body. Optionally, the angular orientation of the detector heads can
be varied either in pairs or individually. Further optionally, one or more of the
heads that are connectable to the same arm can be angularly oriented independently
or differently than other heads.
[0169] According to some embodiments of the invention, at least some of the detector heads
are angularly adjustable to a desired orientation in a plane perpendicular to a longitudinal
axis of the individual detectors. (This feature is generally referred to below as
"rotation" relative to the longitudinal axis.) Typically, but not necessarily, depending
on the shape of the detector head, the longitudinal axis corresponds to an axis of
extension and retraction.
[0170] Optionally, the rotational orientation can be varied from a rest position by up to
90 degrees (or more) such that for some angles and detector dimensions, some or all
the detector heads overlap. This may not only facilitate extending at least some of
the detectors to obtain a smaller bore size, but may also result in obtaining good
360 degree coverage with a smaller number of detectors.
[0171] According to some embodiments of the invention, at least some of the detector heads
are angularly adjustable to a desired orientation that is not in a plane perpendicular
to a longitudinal axis of the detector heads. (This feature is generally referred
to below as "tilting" relative to the longitudinal axis.) Optionally, the desired
tilt angle is achieved by rotation around an axis parallel to an axis of elongation
of the overall system.
[0172] According to some embodiments of the invention, increased reduction in bore size
is achieved by axially spacing the detector heads on the gantry. Optionally, the detector
heads are axially spaced on one side of a single ring on the gantry. Alternatively,
the detector heads are arranged on opposite sides of a single ring. Alternatively
the detector heads are arranged on one or two sides of two separately spaced rings
comprised in the gantry.
[0173] Optionally, for example, in the case of a PET system, adjacent detector head pairs
are located at different axial spacing to avoid interference between adjacent detector
heads. Extension in combination with one of the orientation options may allow a reduced
number of detector heads and/or detector head-pairs in the detector array while still
providing good 360 degree coverage. This can significantly reduce the cost of the
detector array. In addition, reducing the number of detector pairs allows the gantry
to be constructed with open spaces between the detectors along the periphery of the
gantry, which facilitates upgradability as described below.
[0174] It should also be noted that extension of the detector heads to create a smaller
bore size generally has the effect of positioning the detector heads closer to the
ROI, consequently, each detector head subtends a larger solid angle around the ROI,
and is able to collect more photons emitted from the ROI. The result is that overall
system sensitivity may be improved and/or a smaller number and/o size o detectors
may be used. Such approaching is optionally used in PET and/or in non-tomographic
imaging modes, such as planar imaging.
[0175] Positioning the detector heads closer to the ROI may also improve the spatial resolution
by decreasing nonlinearity as discussed below.
[0176] An aspect of some embodiments of the invention resides in N-M tomography systems
in which the detector heads include detector positioning arrangements that are operable
to extend and retract and to angularly orient desired combinations of detectors. Optionally,
the positioning arrangements are comprised of a first mechanism associated with each
detector head to effect extension and retraction, and a separate mechanism to angularly
orient the detector heads. Optionally, a single mechanism for extending and retracting
and angularly orienting the detector heads is associated with each of the detector
heads.
[0177] An aspect of some embodiments of the invention resides in N-M tomography systems
in which the individual detector heads are translatable, e.g., movable laterally or
circumferentially on the gantry, continuously during the scan and/or in steps so that
the spacing between the detector heads can be changed. For example, each detector
head can be translated 5, 10, 15, or 20 degrees, or a greater or lesser or intermediate
amounts from a nominal equally spaced configuration. Optionally, each detector head
is movable independently from the others, or jointly with one or some or all of the
others. Optionally, in combined PET - SPECT dual function systems, either the PET
and SPECT detector heads, or both are circumferentially movable. Optionally, the PET
and SPECT detectors are located at (and/or attached to the gantry at) different axial
positions, for example, on one or both sides of a single rotor disc, or on separate
rotor discs, optionally to provide mechanical clearance.
[0178] As used herein, the term "circumferential movement" refers to rotation of the gantry
ring or rings, and also includes movement of the detector heads on the gantry. Likewise,
circumferential movement includes translational movement of each of the detector heads
individually, i.e., independently, or in groups within the ring, and/or movement of
an entire ring relative to other rings.
[0179] Translation of the detector heads can be advantageous in various situations. For
example, on a gantry having a small number of detector heads e.g., as purchased by
a customer with limited resources, there may be large gaps between detector heads.
Similarly, when the system is constructed of a segmented gantry (optionally with each
segment including more than one detector head) the segments can be moved radially
outward so the bore is expandable when necessary (e.g. to accommodate an obese patient).
In either case, the gaps between the heads may degrade data acquisition. Circumferential
movement effectively shifts the gaps and allows capturing data from the gaps to complete
the missing views.
[0180] Optionally, according to some embodiments, the gaps can be closed by rotation of
the gantry or alternatively by relative rotation among the detectors, for example,
by translating the detector heads circumferentially on a single-ring gantry, of in
the case of a multiple-ring gantry, by rotating one or more of the rings relative
to the others, to complete the full set of angles between pairs of detectors. Effectively,
the bore is expanded radially and gaps are created, the circumferential motion (if
any) allows the gaps to be filled. Optionally, the system is constructed so translation
can occur during a scan or in steps for step and shoot operation.
[0181] In some embodiments, gaps (e.g., between 1 and 30 degrees, for example, between 2
and 10 or 20 degrees) in an axial direction and/or in a circumferential direction
are tolerated. Optionally, reconstruction weights (e.g., for sensitivity) certain
directions according to the presence and/or size of gaps therein.
[0182] A feature of some embodiments of N-M tomography systems according to the present
invention resides in a gantry that is slidable and/or rotatable laterally, to capture
an image from a "body slice" which is orthogonal or not orthogonal to the main body-axis,
and/or move along the body of the patient, for example to capture "slice by slice".
[0183] In an exemplary embodiment of the invention, a controller can selectively move one
or more of the detector heads alone, independently, in groups and/or separately but
optionally in synchrony with other detector heads.
[0184] An aspect of some embodiments of N-M tomography systems according to the present
invention resides in matching the detector bore to the ROI by making the extension
and/or angular orientation adjustable while the scan is being performed.
[0185] A related aspect of some embodiments resides in detector arrays per se having detectors
that are extendable and/or angularly adjustable during a scan.
[0186] By way of summary, adjustment of the size and shape of the bore as well as improved
sensitivity and resolution is achieved by optionally providing one or more of the
degrees of freedom listed below.
[0187] For the gantry (e.g., in either SPECT or PET operation unless otherwise noted):
- (a) The gantry can be a full circle, or a partial circle.
- (b) The gantry can include one ring or multiple rings. The planes defined by the rings
may be parallel to each other, or non-parallel. In a PET mode, the gantry may be rotated
circumferentially, either continuously during generation of data for a slice, or in
steps (referred to herein as "step and shoot" operation).
- (c) The gantry may move vertically relative to the system axis, or can be tilted (e.g.,
using a motor and/or a gear) to one or more non-vertical orientations, and/or to one
or more orientations that are non-orthogonal to the system axis to obtain views that
can overcome attenuation or other obstruction or scatter, or to obtain additional
complementary information that helps stabilize the image reconstruction process.
- (d) Optimally, some or all the adjustments mentioned above can be performed manually.
Optionally or alternatively these adjustments can be motorized and controlled by the
system controller.
For the Detector Heads (e.g., in SPECT or PET operation):
- (e) Some or all the heads can move in and out, radially, i.e. extend and retract,
to increase or decrease the bore size. The extension/retraction can be the same for
all the detector heads, or may be different depending on the location of a particular
ROI in the body;
- (f) The detector heads can move laterally relative to each other and/or to the gantry.
The movement of the detector heads can be linear or along a non-straight-line path,
for example, a curved or piece-wise linear paths elected to avoid or reduce collisions
between adjacent detector heads;
- (g) The detector heads can be rotated around an axis which is substantially orthogonal
to the system axis, e.g., around the axis of extension/retraction;
- (h) The detector heads can be tilted in one or more planes relative to the axis of
extension/retraction. Tilting can be effected by rotating the head around an axis
which is substantially parallel to the system axis, or by rotation around an axis
which is non- parallel to the system axis;
- (i) The system controller can be programmed to move the detector heads in a manner
that prevents collision during movement, for example, by calculating dynamics to predict
a collision and slow down movement as needed. Optionally or alternatively, sensors
are provide (e.g., IR or ultrasonic proximity sensors) at the sides of the detectors,
to detect imminent collisions.
[0188] An aspect of some embodiments of the invention resides in PET systems or in dual
purpose systems operating in a PET mode, in which the detector heads are arranged
around less than the full 360 degrees of the gantry and in which the gantry rotates,
e.g., as in typical SPECT systems. Optionally, the scan is performed at a succession
of axial slices.
[0189] Optionally, the rotation is continuous at each axial position. Alternatively, the
gantry rotates in steps of less than 360 degrees and is temporarily stationary at
each step. Optionally the size of the steps is based on the size of the gaps between
the detectors. Optionally, the continuous or step-wise gantry rotation during a PET
scan is repeated for each of a succession of axial slice positions. Optionally, in
embodiments in which PET detectors are mounted on more than one axially spaced rotor
on the gantry, the rotational speed of each rotor may be the same or different.
[0190] An aspect of some embodiments of N-M tomography systems according to the present
invention resides in the detector arrays being positionable at one or more desired
distances from the patient's body. Optionally, positioning may be done before or during
a scan, optionally continuously, or between axial slices.
[0191] An aspect of some embodiments of the present invention resides in N-M tomography
systems that include proximity detection capability to prevent contact between the
detector array and the body of the patient. Optionally, proximity detection capability
is provided by contact sensors, or by acoustic sensors, or by IR sensors, or by optical
sensors.
[0192] An aspect of some embodiments of the present invention resides in N-M tomography
systems that include proximity detection capability to prevent contact between a detector
and an adjacent detector and/or to prevent contact and/or pinching of body parts between
detectors (e.g., if patient moves his harm into harm's way, for example, proximity
detection capability is provided by contact sensors, or by acoustic sensors, or by
IR sensors, or by optical sensors.
[0193] In an exemplary embodiment of the invention, contact sensors are acceptable, because
detector motion uses low forces, detectors are low-weight and/or covered with a soft
layer and/or detectors can be quickly stopped (e.g., using brakes or suitable motor/actuator
action).
[0194] An aspect of some embodiments of N-M tomography systems according to the present
invention resides in detector arrays that are allowed to make contact with the patient's
body, but with such a low contact force and/or velocity that injury to the patent
does not occur. In some such embodiments, the detector arrays are counterbalanced
on the linear actuator arms so the force needed to extend the detector arrays is acceptably
small (e.g., using a stepper motor which generates up to 3Kg force only). Optionally,
the extension force is small enough that the patient can move the detector array away
from his or her body. Optionally, the actuator allows such back driving, for example,
using gears which can be back driven or by a linear actuator which can be overridden
by patient applied force. Further, because of the small mass of the individual detector
heads, impact with the body is optionally small. Also because of the low mass, the
velocity is easily reduced before impact.
[0195] An aspect of some embodiments of the present invention resides in N-M tomography
systems having modular and/or scalable detector arrays. A related aspect of some embodiments
of the invention resides in modular or scalable detector arrays per se.
[0196] Modularity can allow initial assembly of N-M tomography systems having detector arrays
with a desired number of individual detector heads according to a particular customer's
initial needs, and facilitates subsequent upgrading. This can give a customer the
option, both at the time the system is acquired, and/or at the time of an upgrade,
to trade off cost versus quality, for example, as described herein. For example, three,
four, six, eight, twelve, or an intermediate or greater number of detector heads can
be provided initially, and more added later as needs and/or financial resources of
the customer change. Optionally or alternatively, detectors can be replaced with different
and/or better detectors.
[0197] In an exemplary embodiment of the invention, either by way of identifying data provided
by the added detector heads, or by information provided manually to the system controller,
the software knows what detectors have been installed, and the information can be
used in the course of data acquisition and/or image reconstruction.
[0198] In some embodiments, the detector arrays as originally assembled are for single-mode
SPECT or PET systems. Optionally, the detector arrays as originally assembled include
both SPECT and PET detector heads allowing dual-mode system functionality.
[0199] Optionally, as part of an upgrade, existing detectors may be replaced by better or
improved detectors, for example, having faster circuitry, larger detection area, and/or
better energy and/or spatial resolution. Optionally, detectors can be added and/or
replaced when upgrading either a single-mode or a dual-mode system to improve the
functionality of the system.
[0200] Optionally, an upgrade can convert a single-mode system into a dual-mode system.
Optionally, x-ray CT capability may also be provided in new and/or upgraded single
and dual-mode systems.
[0201] Optionally, features contributing to modularity according to some embodiments include,
without limitation, one or more of the following:
- (a) The gantry which carries the detector array is rotatably mounted in the initially
assembled system, whether it is single-mode (SPECT or PET) or dual mode;
- (b) Connection of the detectors to the coincidence processing electronics is through
a rotatable coupling arrangement;
- (c) The image reconstruction electronics and/or the coincidence processing electronics
are adapted to recognize the number and type (SPECT or PET) of detector heads in the
detector array, either automatically, or by programming at the time of assembly or
upgrading of an existing system;
- (d) The detector heads provide identification as to connection and/or type for auto-recognition
by the electronics sub-systems;
- (e) A rotary drive system is easily installed as part of an upgrade;
- (f) The electronic sub-systems are modular to facilitate converting a single-mode
system into a dual mode system.
[0202] Various ways to implement some of the above-described features will be apparent to
those skilled in the art, especially in view of the exemplary methods of implementations
are described below.
[0203] In some embodiments of the invention, PET detectors are used, i.e., that are used
for detecting high energy photon pairs travelling in opposite directions by identifying
the locations in which 2 photons hit 2 detectors simultaneously (up to photon travel
time and detection time), thus enabling identifying the orientation from which the
photon has been emitted at a much finer precision. For example, such embodiments allow
detection along a line with a width of about 4-6 mm, taking into account the distance
of the positron traveling until annihilated (about 2-4 mm), and the pixel width in
each detector (for example 2-3 mm).
[0204] Optionally, however, in some embodiments, acquisition of PET (high energy) photons
can be done without coincidence detection using "SPECT methodology" (detecting each
photon separately) by providing a thick collimators and detector heads capable of
detecting typical PET and SPECT photons.
[0205] Furthermore, coincidence detection circuitry can optionally include time-of-flight
analysis circuitry to determine where along the estimated emission line the positron
was emitted, for example at a longitudinal resolution of about 1-5 cm, for example
2-3 cm. For example, an optional system clock can be shared by the detector heads.
As an optional alternative, processing is in central location which pre-calibrates
travel time from each detector of signals.
[0206] In some embodiments, the electronic circuitry connected to some or all of the detector
heads includes one or more of the following optional capabilities:
- (a) Photon characterization by energy level (for example, within all the range between
40 Kev and 511 Kev or more);
- (b) Detection time with resolution sufficient to determine coincidence with photon
detection in another detectors,
- (c) Detection time with resolution sufficient to determine time of flight for obtaining
high longitudinal resolution along the detected coincidence line;
- (d) Detection of count rate in case of high flux of photons, for example when a high
intensity radiation source is activated such as X-ray source;
- (e) Optionally, the electronics include multiple separate channels that allow independent
amplification and front-end processing for each detector or small group of detectors
(e.g., 1-5 detectors) and/or a small number of pixels (e.g., between 10 and 1000,
for example 100 pixels). A potential advantage is that malfunction of one or more
pixels or detectors and/or blinding of one or more pixels or detectors by a "hot spot"
(high intensity source) desirably will not prevent other detectors from properly function
and detect photons emitted from other regions, for example as described in US patent publication 2008-0230702-A1.
- (f) Optionally, the processing channels may also be modular, for example, being field
replaceable and/or include their own housings.
[0207] An aspect of some embodiments of the invention pertains to a method of using N-M
tomography systems including detector arrays having some or all the adjustability
features described herein that involves preparing the patient in the normal manner,
setting up the system for an examination by adjusting the bore size and/or shape,
then axially scanning the region of interest, optionally axially. Optionally the adjustment
is achieved by extending at least some of the detector heads and, if necessary, angularly
orienting at least some of the detector heads in the detector array according to the
size and/or shape of the ROI and/or the axial position of the ROI along the body of
the patient. Optionally, the angular orientation is adjusted by rotation of at least
parts of some of the detector heads. Optionally, the angular orientation is adjusted
by tilting at least some of the detector heads.
[0208] Optionally the adjustments can be made during scanning, for example in response to
change in body cross-section, imaging mode.
[0209] Optionally, the method applies to single and dual-purpose systems.
[0210] In a system providing dual-mode functionality, the method further optionally includes
selectable operating the system in a SPECT or in a PET mode.
[0211] In some embodiments, the method involves providing a plurality of additional detector
units that include built-in mechanisms for extension/retraction and angular orientation.
Optionally, the additional detector units are mounted in alternating relationship
with the existing detector units on the gantry. Optionally, if the pre-existing system
does not provide automatic detection of the number and type of detector units, the
method further includes adding automatic detection or programming the emission detector
subsystem according to the number and type of detector units in the upgraded system.
[0212] Optionally, the position adjusting arrangement provided is operable to extend and
retract the detector units, and/or to alter the angular orientation of the detector
units by rotation and/or tilting at least some of the detector units. Optionally,
the position adjusting arrangement includes separate extension/retraction and angular
orientation mechanisms. Optionally, each added detector unit includes its own mechanisms
for extension and retraction, and/or for rotating or tilting.
[0213] Optionally, the detector units of the upgraded system are mounted so that some of
them in the upgraded detector array are axially spaced from others, but on one side
of a gantry ring. Optionally, some of the detector units in the upgraded detector
array are mounted on opposite sides of a detector carrier ring. Optionally some of
the detector units in the upgraded system are mounted on axially offset detector carrier
rings.
[0214] It should be understood, that upgradability as described herein is feasible in systems
that do not contain scalable detector arrays, but the benefits may be attenuated since
the entire preexisting detector array may need to be replaced, and the emission processing
and/or image reconstruction sub-systems may have to be reprogrammed or even replaced.
[0215] An aspect of some embodiments of the present invention resides in N-M tomography
systems in which the detector units are capable of responding to photons in a range
of energies including both PET and SPECT ranges. Optionally, the detectors and associated
emission data processing systems are selectably responsive to PET or SPECT photons,
or, simultaneously responsive to PET and SPECT photons to generate or reconstruct
visual 3D images of regions of interest (ROI) of a patient being examined.
[0216] In such embodiments, and also in other exemplary embodiments described herein, parts
of the data processing systems are optionally contained within or mounted on the detector
units.
[0217] In exemplary embodiments described herein, the radioactive emission detector is comprised
of a scintillator optically coupled to an array of photomultipliers. Alternatively,
the detector is a direct conversion semiconductor array, or a silicon photomultiplier
(SiPM) (see:
Roncali et al., supra).
[0218] Optionally, in the exemplary embodiments described herein, the emission detector
elements are pixilated. Alternatively, at least some detector elements are non-pixilated.
[0219] Optionally, in the exemplary embodiments described herein, the emission detector
elements are formed of a known material including, but not limited to, Lutetium Oxyothosilicate
(LSO), Lutetium Yittrium Oxyothosilicate (LYSO), Cadmium Zinc Telluride (CZT), , Cadmium
Telluride (CdTe), Cesium Telluride (CsTe), Cesium Iodide (Csl), or of any other suitable
and desired material presently known or hereafter discovered or created.
[0220] An aspect of some embodiments of the present invention resides in detector units
including collimators that permit selectable operation in either a PET or SPECT mode,
optionally without physical reconfiguration thereof. Optionally, the detector units
are operable to simultaneously produce PET and SPECT images.
[0221] Optionally, in such embodiments and in other exemplary embodiments described herein,
the collimators are formed of a material that effectively blocks photons having energy
in the range used for SPECT imaging, but is relatively transparent to photons having
energy in the range used for PET imaging. Optionally, the material forming the collimators
blocks no more than about 50% of incident PET photons. Optionally, the collimators
are formed of Tungsten, or Tungsten Carbide or Lead or Gold or depleted Uranium or
a combination of these materials. Optionally, the amount of blocking for PET detection
is selected so that at angles or directions where a higher sensitivity is desired,
there is less blocking.
[0222] An aspect of some embodiments of the present invention resides in detector units
including collimators having adjustable geometry that permits changing the spatial
resolution of the detectors. Optionally, such adjustment permits the detector units
to be selectably operated either as PET or SPECT detectors, or optionally to simultaneously
produce PET and SPECT images.
[0223] Optionally, according to some embodiments, the collimator geometry can be varied
in one or more of the following ways:
- a) increasing the length of the septa forming the collimator cells (the term "length"
referring to the height of the collimator perpendicular to the plane of the detector
module);
- b) increasing the spacing of the septa (i.e., the pitch) in one and/or both directions
relative to a surface of the detector element (optionally, by removing one or more
septa);
- c) tilting some of the septa in one and/or both directions relative to a surface of
the plane of the detector element;
- d) increasing or decreasing the pitch of the septa (i.e. the distance between adjacent
septa walls) in one and or both directions relative to a surface of the detector element:
the pitch can be decreased, for example, by forming the collimator of two or more
relatively moveable parts parallel to the plane of the detector element;
- e) forming the collimator with a shutter to adjust the effective size of the area
exposed to incoming photons: optionally the shutter is slidable or tiltable or in
the form of an iris.
Optionally, the detector element has a planar surface relative to which the septa
are moveable.
[0224] In some embodiments, these modifications are carried out while the collimator is
attached to the detector. In some embodiments, the collimator is removed, modified
and reattached. In some embodiments, the reconfiguration is provided in a laboratory
and/or during manufacture. Optionally, the collimator-detector pair is pre-configured
at multiple collimator states.
[0225] In an exemplary embodiment of the invention, a pressure clamp and/or a screw clamp
and/or a locking rod (through the septa) mechanism are used to hold septa in place
relative to a body of the collimator.
[0226] An aspect of some embodiments of the present invention resides in detector units
including collimators that have a first set of leaves in a first arrangement and a
second set of leaves arranged to intersect with the first set. Optionally, an average
height perpendicular to a detector surface and/or an average thickness of leaves of
the first and second sets are different. This may result in different viewing angles
in different direction and/or different amount of PET sensitivity in different directions.
[0227] An aspect of some embodiments of the present invention resides in a method of imaging
comprising using an N-M tomography system to collect PET and/or SPECT from a ROI using
a single set of detectors. Optionally, PET or SPECT data are collected simultaneously.
Optionally, when PET and SPECT data are collected simultaneously, PET and SPECT images
are generated simultaneously using separate electronic subsystems.
[0228] In an exemplary embodiment of the invention, the amount of axial, circumferential
and/or radial overlap at least 5%, 10%, 20%, 30% or intermediate or greater percentage
of the dimension and/or detector area of the detector head. Optionally or alternatively,
the overlap is less than 90%, 80%, 50%, 40% or smaller or intermediate percentages
thereof.
[0229] Rotation around an axis of a detector can be for example, 10, 30, 40, 70 or smaller
or intermediate degrees.
Optional Features of Some Embodiments of the Invention:
[0230] The discussion below concerns further optional features of some embodiments of the
invention according to the aspects of the invention discussed above. It should be
understood that one or more of these features may be combined with any embodiments
of the detector units and methods described herein above and/or below and/or provided
with other systems, unless otherwise clearly stated:
a. Multiple detector heads arranged around a gantry which are moveable relative to
the patient carrier and/or to each other and/or to the gantry to create a variable-geometry
bore over a wide range of sizes without obstruction or collision of adjacent detector
heads.
b. Adjustability of the bore geometry during a scan, optionally between steps of a
step-and-shoot scan, or between axial positioning of the gantry for acquisition of
data for a succession of axial slices. Optionally, adjustment may be performed both
between step-and-shoot positions and between axial positions, or "on the fly" according
to a pre-set program for a specific patient scan, both during scan or in a sequences
scan scenario.
c. Rapid reconfiguration of detector geometry facilitated by light-weight design of
the detector heads and/or by counterbalancing the detector units.
d. Variable bore geometry implemented in conventionally configured systems (with the
patient lying on a horizontal carrier) and also systems, in which the patient stands
or sits such that system axis (as well as the main patient axis) is vertical, and
the gantry is relatively horizontal.
e. PET detector heads that are T-shaped, or L-shaped, and SPECT detector heads that
are rod or I-shaped.
f. Detector heads including elongated stems that serve as an axis for extension/retraction
with the detector elements arrayed on the ends of the stems in various polygonal configurations
including square or rectangular or triangular, or in circular or arc-shaped configurations,
or combination thereof. Optionally, in rest positions, the detector elements extend
longitudinally in the direction of the system axis.
g. Detector heads that are comprised of a single detector element or of a plurality
of detector elements, for example, pixilated detectors. For convenience, the terms
"detector arrangement" or "detector system" will sometimes be used in reference to
multiple and single-detector heads, and to heads that carry SPECT and/or PET detectors,
and without distinction as to detector head shape.
h. Variable-geometry detector systems in which the detector units are non-uniformly
arranged on a gantry with large gaps between them wherein the adjustability of the
detector heads still provides full 360 degree detector coverage without loss of sensitivity
and spatial resolution for differently sized and shaped ROIs and at different positions
along the body of a subject under examination. In some embodiments of the present
invention, such configurations possibly reduce the overall number of detectors needed
for a given level of spatial resolution and sensitivity, and potentially reduce the
overall system cost.
i. Detector heads that are extended and retracted by linear actuators. Optionally,
in a PET mode, detector heads that are extended individually or in opposed pairs.
Optionally, the opposed pairs are diametrically opposed. Optionally, multiple detector
heads are mounted on and/or moved together along a single arm for in-out and/or lateral
motion.
j. Detector arrays in which some of the detector heads are not moved and data for
imaging is optionally collected from both moved and unmoved detector heads.
k. Detector heads that include electronic circuitry that supports more than one set
of separate detector elements, e.g., of different types and/or geometric location.
l. Variable geometry detector arrays in which the detector heads; (particularly but
not exclusively in the case of PET detector heads) are moveable in ways that permit
bores small enough for efficient imaging of relatively small organs such as the brain,
the throat or an extremity, without collision or interference between adjacent detector
heads. Options include:
- (i) making only some of the detector heads, for example, every other detector head
extensible, (with the un-extended detector heads optionally used or are not used during
the scan,
- (ii) making the angular orientation of at least some of the detector heads adjustable
relative to the axes of extension of the respective detectors to increase the amount
that the detectors can be extended without collision. Optionally, the angular orientation
of the detector heads can be varied either in pairs or individually. Further optionally,
one or more heads that are connectable to the same arm can be angularly oriented independently
or differently than other heads,
- (iii) making at least some of the detector heads rotatable or tiltable. It should
also be noted that extension of the detector heads to create a smaller bore size has
the effect of positioning the detector heads closer to the ROI, consequently, each
detector head subtends a larger solid angle around the ROI, and is able to collect
more photons emitted from the ROI, The result is that overall system sensitivity may
be improved.
m. making detector heads translatable, i.e., movable circumferentially on the gantry,
continuously during the scan or in steps so that the spacing between the detector
heads can be changed. For example, each detector head can be translated 5, 10, 15,
or 20 degrees, or a greater or lesser or intermediate amounts from a nominal equally
spaced configuration. Optionally, each detector head is movable independently from
the others, or jointly with one or some or all of the others. Optionally, in combined
PET - SPECT dual function systems, either the PET and SPECT detector heads, or both
are circumferentially movable. Optionally, the detectors heads can be moved in one
or more straight line segments or along a curve.
n. Locating translatable PET and SPECT detectors at different axial positions, for
example, on one or both sides of a single rotor disc, or on separate rotor discs to
provide mechanical clearance.
o. Configuring the detector arrays so that gaps between then can be effectively closed
by rotation of the gantry. (As used herein, the term "circumferential movement" also
refers to rotation of the gantry ring or rings, continuously and/or in steps) as well
as by translating the detector heads circumferentially on the gantry to complete the
full set of angles between adjacent detector heads. Optionally, the system is constructed
so translation can occur during a scan or in circumferential steps (for step and shoot
operation) or between axial positions.
p. Making the gantry slidable and/or rotatable laterally, to capture an image from
a "body slice" which is orthogonal or not orthogonal to the main body-axis, and/or
move along the body of the patient, for example to capture "slice by slice".
q. Providing for selective movement of one or more the detector heads alone, independently,
in groups and/or separately but in synchrony with other detector heads.
r. The gantry can be fully circular, or a partial circle, as well as other shapes.
s. The planes defined by multiple gantry rings may be parallel to each other, or non-parallel.
t. The gantry may move vertically relative to the system axis, or can be tilted to
one or more non-vertical orientations (e.g., be mounted on a motorized axle), and/or
to one or more orientations that are non-orthogonal to the system axis to obtain views
that can overcome attenuation or other obstruction or scatter, or to obtain additional
complementary information that helps stabilize the image reconstruction process.
u. Gantry rotation is continuous at each axial position for both PET and SPECT imaging.
Alternatively, the gantry rotates in steps, the size of which is optionally based
on the size of the gaps between the detectors. Optionally, in embodiments in which
PET detectors are mounted on more than one axially spaced rotor on the gantry, the
rotational speed of each rotor may be the same or different.
v. Providing proximity and/or side detection capability to prevent contact between
the detector array and the body of the patient. Optionally, proximity detection capability
is provided by contact sensors, or by acoustic sensors, or by IR sensors, or by optical
sensors, or in any other suitable and desired manner.
w. Permitting the detector heads to make contact with the patient's body, but with
such a low contact force and/or velocity that injury to the patent does not occur.
x. Counterbalancing the detector heads so the force needed to extend the detector
heads is acceptably and safely small. Optionally, the force is small enough so that
a patient can easily resist the force or can move the detector array away from his
or her body by hand if necessary. The optional small effective mass of the individual
detector heads, allows the velocity to be easily reduced before impact.
y. The detector head counterbalancing mechanisms include adaptive motion feedback
capability for safety control and acquisition continuation if the detector head is
touched or pushed back, for example, by the patient.
z. Configuring the system so that the detector arrays are modular or scalable.
aa. Either by way of identifying data provided by the detector heads added as part
of an upgrade, or by information provided manually to the system controller, the system
software is made aware of what detectors have been installed, and the information
can be used in the course of data acquisition and image reconstruction.
bb. The gantry which carries the detector array may be rotatably mounted in the initially
assembled system, whether it is single-mode (SPECT or PET) or dual mode;
cc. The detector heads are connected to the processing electronics through a rotatable
coupling arrangement;
dd. The gantry is configured so it requires only minimum disassembly for upgrading,
including to facilitate installation of a rotary drive system, thereby helping to
permit on-site upgrading;
ee. The electronic sub-systems are modular to facilitate converting a single-mode
system to a dual mode system.
ff. Coincidence detection circuitry for PET imaging can include time-of-flight analysis
circuitry to determine where along the estimated emission line the positron was emitted,
for example at a longitudinal resolution of about 1-5 cm, for example 2-3 cm. For
example, an optional system clock can be shared by the detector heads. As an optional
alternative, processing is in central location which pre-calibrates travel time from
each detector of signals.
gg. The electronic circuitry connected to some or all of the detector heads includes
one or more of the following optional capabilities:
- (i) Photon characterization by energy level (for example, within all the range between
40 Kev and 511 Kev);
- (ii) Detection time resolution sufficient to determine coincidence with photon detection
in another detector,
- (iii) Detection time resolution sufficient to determine time of flight for obtaining
high longitudinal resolution along the detected coincidence line,
- (iv) Detection of count rate in case of high flux of photons, for example when a high
intensity radiation source is activated such as X-ray source.
hh. The electronics include multiple separate channels that allow independent amplification
and front-end processing for each detector or small group of detectors and/or a small
number of pixels (e.g., between 10 and 1000, for example 100), such that any malfunction
of one or more pixels or detectors and any blinding of one or more pixels or detectors
by a "hot spot" (high intensity source) do not prevent other detectors from properly
function and detect photons emitted from other regions. Optionally, the processing
channels may also be modular.
[0231] An aspect of some embodiments of the invention resides pertains to a method of using
an N-M tomography system including detector arrays optionally having some or all the
adjustability features described herein. In some embodiments, both PET and SPECT imaging
can be performed, either sequentially or simultaneously. Optionally, the method involves
preparing the patient in the normal manner, setting up the system for an examination
by adjusting the bore size and/or shape, then scanning the ROI. Optionally the adjustment
is achieved by extending at least some of the detector heads and, if necessary, angularly
orienting at least some of the detector heads in the detector array according to the
size and/or shape of the ROI and/or the axial position of the ROI along the body of
the patient.
[0232] In a system providing CT capability, the latter modality may optionally also be employed
as part of a unified examination,
[0233] An aspect of some embodiments of N-M tomography systems according to the present
invention resides in use of collimated detectors for PET imaging, with image reconstruction
software that compensates for reduced off-axis sensitivity resulting from photon absorption
by the collimator septa, for example, by weighting of photon counts according to their
direction of impact.
Exemplary System Features:
[0234] Referring again to Fig. 1A, in some exemplary embodiments of the invention, gantry
12 is mounted so that in addition to conventional functionality by which it moves
along the length of patient carrier 16 (or
vice versa) to capture emission data from a succession of "slices" orthogonal to the length of
the patient's body, (i.e., the body-axis), it is optionally also constructed so it
can slide transversely (e.g., on a rail) or tilt relative to the body-axis (e.g.,
the rail is mounted on an axle or an actuator is provided at either end to raise/lower
a side of the rail), to capture emission data in planes that are not orthogonal to
the body-axis.
[0235] This capability can be advantageous, for example, if it is desired to acquire photons
from viewing angles with less attenuation and scatter due to bones (e.g. taking different
viewing angles to overcome attenuation and scatter by the ribs, etc), or to improve
uniformity, quality and stability of the image reconstruction process by providing
to the reconstruction algorithm information from additional viewing angles.
Modularity and Upgrade:
[0236] An aspect of some embodiments of the invention is modularity of the detector arrays.
Referring now to Figs. 3A - 3D, several implications of this are described in the
context of a detector array 300 for a SPECT system according to some embodiments of
the invention. In the illustrated context, it should be understood that modularity
applies to the design of detector array 300 such that it may be assembled from a desired
number of individual detector heads 302a, 302b, etc. according to the specification
of the customer.
[0237] Fig. 3A illustrates a detector array 300 with three individual detector heads 30
(two being a practical lower limit in conventional systems). Figs. 3B-3D respectively
illustrate systems employing detector arrays having four, six and 12 individual detector
heads 302. Exemplary configurations of detector heads embodying features of the present
invention are described below.
[0238] Figs. 3A-3D also illustrate the trade-off resulting when increasing numbers of detector
heads are provided: performance is increased in terms spatial resolution and/or sensitivity
and/or speed of image data acquisition for a range of ROI sizes and shapes and longitudinal
(axial) locations along the body of the subject of an examination, but at potentially
significant increased cost for the detector heads.
[0239] Another implication of modularity is illustrated in Figs. 4A and 4B: a system can
initially be assembled with a detector array 400 providing single-mode functionality
(here illustrated as SPECT functionality) and can conveniently be upgraded into a
dual-mode system that provides both SPECT and PET functionality.
[0240] Thus, Fig. 4A shows an as-built detector array 400 for a SPECT system having six
detector heads 402 with considerable open space 404 between the individual detectors.
Fig. 4B shows a detector array 406, for example, after an upgrade of detector array
400. Here, six PET detector heads 408 have been installed in alternating relationship
with SPECT detector heads 402. As will be understood, Fig. 4B can represent an as-built
configuration or an upgraded detector array comprised only of 12 PET detectors 408
or only of 12 SPECT detectors 402.
[0241] In an exemplary embodiment of the invention, a modular attachable/detachable component
includes a housing suitable for exterior viewing/environment, for example, with suitable
paint and/or markings. In an exemplary embodiment of the invention, the attachment
of the component to the rest of the system includes separate electrical, data and
mechanical connectors. For example, plug-socket connectors may be used for power and
data and a mechanical interlock used for mechanical connection. In some embodiments,
the component will interlock to a movable part of the system. In an exemplary embodiment
of the invention, two interlocks are used; one interlock providing alignment between
the detector and the system (or gantry), for example, a plurality of pins matching
recesses and/or other geometries and a second interlock provides interference to prevent
retraction, for example, using one or more screws, bolts or a locking rod. Optionally,
a separate element (from the alignment geometry), such as a rectangular rod, is used
to convey forces between the system and a removable detector
Basic Detector Head Configurations
[0242] Fig. 5 shows exemplary details of SPECT and PET detector heads according to some
embodiments of the invention mounted on one side of a single gantry 500. SPECT detector
heads 502 are shown as rod or I-shaped with arcuate, e.g., approximately cylindrical
photon-collecting surfaces 510 extending into the plane of the figure. PET detector
heads 504 are shown as T-shaped with a stem portion 506 extending radially toward
the system axis at the center of gantry 500 and a detector-carrying portion 508 oriented
tangentially to the periphery of the bore.
[0243] It will be appreciated that other external configurations are also within the scope
of some embodiments. For example, both the SPECT and PET detector heads can be L-shaped
or otherwise have different shapes. Optionally, different detectors with different
abilities have different shapes and/or sizes.
[0244] Optionally and preferably, in some embodiments, the detector-carrying portions 508
of PET detector heads 504 are configured as plates that extend in the direction of
the system axis, i.e., into the plane of the figure. This can be advantageous, for
example in that it allows a desired degree of overlap between slices as the emission
data is being collected, or wider slice width, to perform faster body scan (in the
case of multiple-slice scan).
[0245] The large detector head configuration for the PET detector heads 504 can be advantageous
because for optimal and uniform PET image reconstruction, pairs of PET detectors sometimes
need to cover as much as possible of the whole 360 degrees of possible photon emission
from each location in the ROI, and/or with a sufficient axial extent. Having large
detector surfaces for the PET detector heads may minimizes gaps in which coincidence
lines are not covered and/or otherwise increase sensitivity, and may avoid reduced
uniformity and/or sensitivity.
[0246] SPECT detectors, on the other hand can acquire 180 degrees around the ROI in several
positions at different times, so, in some embodiments they can be narrower and move
to obtain the necessary viewing angles. In an exemplary embodiment of the invention,
care is taken, however, that the PET detectors are not so large that they obscure
the view of the SPECT detectors when only the latter are in use.
[0247] As described herein, the dynamically variable geometry of the detector heads according
to some embodiments of the invention facilitates optimizing or near-optimizing the
size and/or shape of both the PET and SPECT detectors. Potentially contributing to
optimization is the placement of the detectors on one or more gantry rings which can
move and operate independently as discussed herein. In an exemplary embodiment of
the invention, the system controller 30 (see Fig. 2) may be programmed to plan and/or
control the motion of the detectors heads according to a desired (e.g., optimal) data
collection from the desired ROI, while optionally preventing adjacent detector heads
from colliding and/or obscuring each other's field of view.
[0248] In some exemplary embodiments, the SPECT detector heads include detectors (e.g.,
radiation sensitive elements thereof) that cover overall about 1-40 cm, or 1-20 cm,
for example, about 2-8 cm along the circumferential dimension, i.e., along the gantry,
for example about 4 cm. In the axial direction, i.e., in the direction of the system
axis, the SPECT detectors optionally cover overall about 10-40 cm, for example about
12-32 cm, or 15-30 cm, or 16-28 cm, or about 16 cm, or about 20 cm, or about 24 cm,
or about 28 cm or intermediate sizes.
[0249] In some exemplary embodiments, collimators extend radially, i.e. toward the patient's
body a distance of a few cm (e.g. 1-20 cm, 1-4 cm, for example about 2-3 cm),
[0250] In some embodiments, the SPECT detectors (with the collimator) of each such detector
head are rotatable, for example, around an axis parallel to the system axis. In some
embodiments, the overall space required to enable such free rotation of the head along
the circumferential dimension can be about 6-15 cm wide, for example 7-12 cm, for
example about 10 cm.
[0251] In an example, the PET detector heads include detectors that cover overall about
2-50 cm along the circumferential dimension, for example about 2-40 cm, or 2-35 cm,
or 3-30 cm, or 5-28 cm, or 10-28 cm, or 15-25 cm, or 20-25 cm, and cover overall about
2-35 cm along the axial dimension, for example about 2-30 cm, or 2-25 cm, or 2.5-20
cm, or 2.5-17 cm, or 2.5-15 cm, or 3-10 cm, or 3-9 cm, or 3-8 cm, or 3-5 cm, for example
less than 15 cm, and optionally less than 10 cm, or for example about 5 to 9 cm, or
for example 7 to 8 cm.
Dynamically Variable Bore Geometry:
[0252] Conventionally, in both PET and SPECT systems, the bore size is not adjustable, and
therefore the sensitivity and/or spatial resolution vary according to the particular
ROI and body location. The conventional solution has been to provide a bore adequate
to accommodate a full-body scan and accept degradation in performance when a smaller
bore would have been preferable for a particular ROI as explained below. Another conventional
solution has been special small-bore systems for brain or neck scans.
[0253] According to some embodiments of the present invention, N-M tomography systems are
provided with a dynamically variable-geometry bore. Several ways to achieve this are
implemented by providing the degrees of freedom for gantry configurations and/or detector
head extension/retraction and/or angular orientation. In an exemplary embodiment of
the invention, these are pre-selectable, i.e., before performance of a scan, and/or
adjustable during the scan, either continuously or in steps.
[0254] Fig. 4B shows a 12 detector head system with alternating PET and SPECT detector heads
on a circular gantry in which the heads are all in a fully retracted configuration
providing a maximum bore size for both PET and SPECT operation. Optionally, a non-one
to one relationship of the number of PET and SPECT is possible.
[0255] In the configuration shown in Fig. 5, all the PET detector heads 504 have been extended
to provide a reduced bore size for PET operation. Alternatively only SPECT detectors
are extended. It may be seen that Fig. 5 represents approximately the maximum extension
possible without collision of adjacent heads to the illustrated size and configuration
of the PET detector heads illustrated.
[0256] Fig. 5 also shows exemplary extension/retraction mechanisms 510 and 512 respectively
for the PET and SPECT detector heads, described more fully below.
[0257] Figs. 6A-6D, illustrate spatial resolution improvement that can be achieved when
the bore size is increased or decreased to take account of the particular ROI and
body location. The example shows a SPECT only system with 12 detector heads 602 on
one side of a single-ring circular gentry 600, but the same benefits can be achieved
in PET only systems, and in systems capable of both SPECT and PET operation.
[0258] Fig. 6A shows detector heads 602 in a retracted configuration to provide a large
bore, for example, a conventional 90 cm. bore, for performing a full body scan. Fig.
6B illustrates detector heads 602 fully extended to provide a small bore, for example,
20 cm., as it would be used, for example, when performing a brain scan or a scan of
the neck.
[0259] It should be appreciated that the cross-section of an ROI will generally not be circular,
particularly in the case of a body scan, so the possibility non-uniform resolution
and/or sensitivity may exist. According to some embodiments of the invention, if desired
(or for other reasons) this can be alleviated in some instances by varying the orientation
of the detection surfaces dynamically during the scan. For the arrangement illustrated
in Figs. 6A and 6B, this can be achieved by rotating the detector-carrying portions
604 of detector heads 602 around an axis parallel to the system axis during a scan.
The detectors can optionally be rotated individually or together. Since such rotation
can effectively fill gaps between adjacent detector heads, it may also allow obtaining
good sensitivity and resolution with a smaller number of detectors, and thereby result
in a less costly system.
[0260] It should also be noted that in an exemplary embodiment of the invention, only the
detector element bearing parts of the detector heads need to be moved. Since these
are not heavy, rapid dynamic changes in orientation are practical.
[0261] One potential effect (which may be beneficial) resulting from matching the bore size
to the ROI being examined is an increase in the acceptance angle for incoming and/or
scattered photons. This is illustrated in Fig. 6C. Here, a small ROI 604 is assumed
to be centered in a bore 606a that is large compared to the ROI, or in a bore 606b
that is closely matched to the size of the ROI. In an exemplary embodiment of the
invention, an emission event 605 is assumed to be centered in ROI at 604. As a result
of scattering the two photons travel along angularly displaced paths: 607a for the
un-scattered photon and 607b for the scattered photon, instead of path 607c. The acceptance
angle α, i.e. the angular error between paths 607b and 607c, is a function of the
bore size and the detector pixel size according to the relationship:
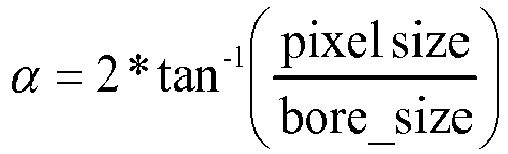
[0262] In an exemplary embodiment of the invention, an acceptance angle α1 for the large
bore can be increased to an angle α2 of, for example, up to about three or four times
without degrading or decreasing resolution by reducing the bore size to match the
ROI.
[0263] Another effect, potentially beneficial which may result from matching the bore size
to the ROI being examined is illustrated in Fig. 6D. This relates to reduction of
the so-called "non-co-linearity effect" resulting from residual momentum of the electron
and positron annihilation. A positron event 609 produces emission of a photon pair
that do not travel in exactly opposite directions. One photon travels along a path
611a instead of 611b and is detected by a detector head 608a instead of 608b, while
the other travels along path 611c and is detected by detector head 608c aligned with
detector head 608b. By decreasing the bore size from 90 cm to 20 cm, the photon on
path 611a is detected by detector head 608b, whereby degradation of resolution due
to non co-linearity can be reduced by a factor of up to, for example, 2 or 3 or more
with a smaller bore size.
[0264] In general, the improvement factor is dependent on the starting and ending bore sizes.
In the illustration it is reduced from 90cm to 20cm. The angle error due to non-colinearity
is about ±0.25 degree. This error is estimated, for example, based on the energy range
of the residual momentum of the electron and positron annihilation. The resolution
degradation correspond to the shift between the theoretical event position (when colinearity
is perfect) to the actual event position. The error can be estimated with simple trigonometry
as Err = tan(alpha) * (Bore_size/2). The error for the 90cm and 20cm bore size can
therefore be reduced by up to about 4.5.
[0265] Adjustment of bore size by extension and/or retraction of the individual detector
heads may be achieved in various ways. A linear motion mechanism can be implemented,
for example, with a DC or AC motor, or linear actuator, by a stepper motor or hydraulically.
Position detectors, for example, limit switches, resettable counters, or digital or
analog encoders, may be used to provide position feedback and/or avoid over extension
and/or collision. The scope of the invention is not necessarily limited by these methods
and other ways will be apparent to those skilled in the art as well.
[0266] Figs. 6E-G illustrate an arrangement generally designated at 620 for extending and
retracting a detector head 622, according to some exemplary embodiments of the invention.
Fig. 6E is a top and side perspective view, Fig. 6F is a side elevation, and Fig.
6G illustrates an exemplary linear actuator.
[0267] Sub-assembly 620 includes a detector head 622 located at one end 624 of an arm 626,
which is movably mounted on a linear rail 628 extending radially on a gantry 630.
Within or (optionally) attached to the outside of arm 626 is a linear actuator arm
634 (Fig. 6G). Control of extension and retraction is provided by system controller
30 (see Fig. 2).
[0268] A weight 632 chosen to balance the weight of detector head 622 and arm 626 is moveably
mounted on rail 628 and attached to arm 626 for example, by a suitable pulley arrangement
and belt or cable or in any other suitable and/or desired manner. For example, the
weight can be between 0.5 Kg and 30 Kg, for example, between 3Kg and 20 Kg, for example,
7 Kg. Optionally, the moving part of the detector weighs between 1 and 30 Kg. Optionally,
the entre arm module weights between 2 and 50 Kg, for example, between 5 and 30 Kg.
[0269] Referring to Fig. 6G, in which some parts are omitted in the interest of clarity,
in the illustrated example, arm 634 includes a driving member 636, for example, a
sprocket, and a driven member 638 which may also be a sprocket. Input power is provided
by a rotary actuator such as a motor described above (not shown) attached to sprocket
634. A chain (not visible in Fig. 6G) is carried by sprockets 634 and 636.
[0270] Attached to the chain are travelers 638 and 640, which respectively carry detector
head 622 and its arm 626, and counterweight 632. As will be appreciated, in an exemplary
embodiment of the invention, when the chain is driven, the detector head and counterweight
move in opposite directions, as indicated by arrows 644 and 646.
[0271] Such an arrangement allows use of a very small force for extension and retraction
while gravitation does not produce any motion or resistance since the counterweight
provides a balancing counter force equal to the projection of the total force (vector)
along the path of linear motion.
[0272] In some exemplary embodiments, for a detector head weighting about 20kg, counter-balance
can be provided by a weight of approximately 19.5kg, so a force of only about 0.5kg
will be needed to be employed for moving the detector head.
[0273] Such a very gentle force potentially reduces risk of patient injury in case of a
collision. Moreover, in case of a collision, the patient can typically easily resist
such gentle force and/or move the arms away regardless of their orientation.
[0274] Additional or alternative collision avoidance protection may be provided by proximity
sensors of various types. Some options include pressure sensors, acoustic, e.g., ultrasound
sensors, and optical sensors mounted on the detector units and coupled to control
the actuator motor in a suitable manner. In one example, a controller receives an
alert when the distance is below a certain threshold. In another example, the motor
is stopped (or a brake activated or motor disengaged) by a dedicated electrical circuit
reading the sensor.
[0275] In some embodiments, the detector heads are brought into close proximity to the patient's
body, e.g., within less than 20 cm, or less than 10 cm, or less than 5 cm or within
1-2 cm or in substantial contact or larger or smaller or intermediate distances.
[0276] Optionally, in some embodiments, contact with the patient's body is allowed, but
the allowable force on the body/skin is limited, for example, to less than 1000 g,
or less than 200 g, or less than 50 g, or less than 10 g or intermediate contact forces.
In an exemplary embodiment of the invention, the contact area is at least 1-10 cm
squared, optionally by having detector not have sharp edges in direction of body.
[0277] In an exemplary embodiment of the invention, consideration is given to assuring that
adjacent heads do not interfere mechanically or operationally. Figs. 7A and 7B, 8A-8E,
9A-9C, 10A and 10B, and 11A-11C illustrate exemplary design solutions according to
some embodiments of the invention.
[0278] Figs. 7A and 7B illustrate a dual-mode system 700 comprised of a detector array 706
comprised of six PET detector heads 702a-702f arranged in three pairs 702a,d, 702b,e,
and 702c,f and six SPECT detectors 704. System 700 is illustrated as being used in
PET mode. In Fig. 7A, The PET detectors comprised in array 706 are in and extended
operating configuration for example suitable for performing full body scans. However,
because of the shape of the PET detectors, Fig. 7A is also about the minimum practical
extension that is achievable due to the size and shape of the detector heads.
[0279] In an exemplary embodiment of the invention, the controller includes a geometry engine
which simulates the space filling properties of the detectors so as to plan and/or
monitor motion in a manner which avoids interference between moving (and/or unmoving
components). Optionally or alternatively, the geometry engine also calculates lines
of sight and/or obstructions. In an alternative embodiment, allowable paths and/or
positions (e.g., for one or more detectors) are pre-calculated and provided to the
system, which uses such paths and/or positions.
[0280] To achieve a smaller bore size with detector array 706, only some, for example one-half
of, or any subgroup of, the detector heads, for example, detector heads 702b, 702d,
and 702f, are extended, as illustrated in Fig. 7B. The other detector heads 702a,
702c, and 702e remain in a fully retracted position. In some embodiments, only the
extended detector heads are used. In other embodiments, all the detector heads are
used, but the sensitivity and/or resolution of the extended detector heads may be
not the same as for the un-extended detector heads. This is optionally accounted for
in the course of processing the emission data.
[0281] Figs. 8A-8D illustrate other ways to achieve smaller bores, one such way, again in
a dual-mode system being operated in a PET mode. Fig. 8A shows the detector element
carrying portions of PET detector heads 806a, 806c, and 806e rotated 90 degrees around
their respective longitudinal axes (i.e., in a plane perpendicular to the longitudinal
axes. Compared with Fig. 7A, it may be seen that a smaller bore is achieved.
[0282] Fig 8B shows a configuration in which all the PET detector heads 802 have been rotated
by 90 degrees, allowing the greatest reduction in bore size.
[0283] Fig 8C represents a situation in which all of detector PET heads 802 have been rotated
by less than 90 degrees, for example, 45 degrees.
[0284] Such rotation may take place, for example before during or after extension, and/or
during a scan as described below.
[0285] Fig. 8C illustrates the effect of rotating the PET detector heads 806a-806f to an
angle between 0 degrees and 90 degrees relative to the orientation in Fig. 8A, followed
by extension. As may be seen from Fig. 8C, such rotation can result in overlap of
adjacent detector heads.
[0286] In some embodiments of the invention, as the angle of rotation increases toward 45
degrees, the overlap increases, but decreases as the angle of rotation increases further
toward 90 degrees, at that point, the limiting case of no overlap is reached, as illustrated
in Fig. 8B.
[0287] The rotation shown in Figs. 8A-8D can be implemented in various ways. For example,
small bidirectional motors (not shown) may be mounted on gantry 814 and connected
through a position tracking arrangement, for example, using encoders and/or limit
switches coupled to detector shafts 808a, etc. Optionally, each detector head unit
may include a built-in rotation actuator. Other mechanical arrangements may also be
used, for example, a hydraulic arrangement, or manual adjustment may be also be employed.
Optionally, for this and/or for actuators for extension and retraction, the motors
are controlled by the system controller.
[0288] Another way of reducing bore size is illustrated in Figs. 9A-9C. Fig. 9A illustrates
a system 900 in which the individual detector heads 902a-902f in a detector array
906 are partially extended, for example, for a full-body PET scan, and also are oriented
in planes perpendicular to the respective axes of elongation of the detectors as in
the other embodiments described up to now. However, it should be noted that the extension
illustrated in Fig. 9A is about the greatest possible extension since attempted further
extension is blocked by peripheral contact between the detector heads.
[0289] In an exemplary embodiment of the invention, to create clearance for further extension
and reduction of the bore size, the detector heads are tilted out of the perpendicular
planes by pivoting them around axes that run parallel (and/or perpendicular and/or
other axes that are in the plane of the detector) to the longitudinal axis of the
system, e.g., in the direction parallel to direction of relative movement by which
the successive axial slices are produced. As a consequence, as illustrated in Fig.
9B, adjacent detector heads, e.g., heads 904a and 904b overlap peripherally allowing
a degree of extension of detectors 902a-902f not achievable with the heads in the
respective perpendicular planes as illustrated in Fig. 9A.
[0290] Optionally, to achieve yet further reduction in bore size, heads 904a- 904f are tilted
even further as illustrated in Fig. 9C thereby increasing the overlap and allowing
additional extension.
[0291] The tilting shown in Figs. 9A-9C can be implemented in various ways similar to those
employed in the embodiments of Figs. 8A-8C, as will be understood by persons skilled
in the art.
[0292] It is noted in these and other embodiments, that the detector plane need not be flat.
For example, it may be curved being a section of a cylinder, a sphere and/or other
conic section and/or other curved and/or piecewise linear shape.
[0293] Figs. 10A and 10B illustrate a detector array 1000 employing a further way of achieving
a wide range of bore sizes, which is optionally used together with other methods as
described herein. Here, detector array 1000 includes six PET detector heads 1002a-1002f
and six SPECT detector heads 1004a-1004f mounted on a gantry ring 1006. To accommodate
the shape of the PET detectors, detector head pairs 1002a, 1002d and 1002c,1002f are
located in a first plane on the gantry, while intervening detector head pairs 1002b,
1002e, are located on a second plane spaced axially from the plane of the other two
pairs.
[0294] Fig. 10A illustrates extension of the PET detectors to achieve a first desired bore
size. To achieve a smaller bore size, the detectors are extended as desired, as shown
in Fig. 10B. Because of the axial spacing, even after the detectors have been extended
sufficiently that they would come in peripheral contact if they were situated in a
single plane, further extension is possible because the axial spacing allows the tip
of one detector, for example 1002a, to pass behind the adjacent tip of the next detector
1002b.
[0295] As will be appreciated, suitable modification of the programming of the coincidence
detection sub-system may be made to account for the axial spacing. In some embodiments
the programming does not need to be modified (but only parameters or look up tables)
since it just needs to note the non-uniform viewing when determining relative counts/normalizing
from different areas (e.g., using sensitivity maps and/or other calibration maps).
[0296] Figs. 11A-11C illustrate another arrangement for achieving a wide range of bore sizes
involving axial spacing, which may be used, for example, alone or with other methods
described herein. Fig. 11A is an end view of assembled detector array 1100. Fig. 11B
is a perspective view with the top portion cut away and Fig. 11C is a perspective
view rotated 90 degrees from that of Fig. 11B to show internal construction details.
[0297] Here, detector array 1100 is comprised of six PET detectors 1102 and six SPECT detector
heads 1104. To accommodate the shape of the PET detectors, detector pairs 1002a, 1002d
and 1002c, 1002f are located on a first ring 1106 of a gantry 1108, while intervening
detector pair 1102b, 1102e, is located on a second ring 1110 spaced axially from the
ring of the other two pairs. Optionally, since ring 1110 carries only one PET detector
pair, all the SPECT detectors may be mounted on that ring.
[0298] Optionally, more than two rings may be provided. Fig. 11D shows an embodiment in
which the detector heads are arranged in three layers, either on one ring or on three
separate rings. Additional rings, for example, 3, 4, 5, 6 or more may be provided
(and optionally added in a modular manner). In an exemplary embodiment of the invention,
the detectors on different rings are of different types, sizes and/or qualities.
Detector Head Movement on the Gantry and Gantry Rotation:
[0299] A potential benefit of the variable geometry aspect of some embodiments of the invention
is the possibility of obtaining good resolution and sensitivity around the entire
ROI with a reduced number of detector units that are laterally or circumferentially
moveable on a gantry. Fig. 12A illustrates schematically one way of translating detector
heads on a gantry. Here, eight detector heads 1202a-1202h are slidably mounted on
a track assembly 1204 comprised of separated track segments 1206a-1206h.
[0300] In an exemplary embodiment of the invention, heads 1202 may be moved along their
respective track segments by a linear motion arrangement similar to that described
in connection with Fig. 6G, or by any other suitable and desired arrangement.
[0301] Various movement options may be provided, as will be apparent to those skilled in
the art in light of the disclosure herein, for example, but not limited to (a) prepositioning
(b) steps of a step and shoot regimen, (c) adjustment of position between axial slices,
and (d) "on the fly" adjustment during gantry rotation or a spiral scan. It should
be recognized that combination of the indicted or other options are also contemplated.
Further it should be recognized that the detector heads may be positioned either uniformly
or non-uniformly around the gantry. Movement over any desired range depending on the
number of detector heads possible, for example, 20 degrees (i.e., ± 10 degrees from
a central position). The described arrangements are applicable to PET and SPECT detector
heads as well as dual purpose heads as described below.
[0302] Fig. 12B illustrates an alternative arrangement in which the detector head move circumferentially
on the gantry. Here, a detector array 1208 mounted on a gantry 1209 includes six detector
heads 1210a-1210f. With respect to at least some designs, the concepts being described
are equally applicable to PET and SPECT detectors. As in other embodiments described,
the detector heads can be extended and/or retracted, and/or optionally rotated or
tilted, to vary the bore size/
[0303] In an exemplary embodiment of the invention, for example, to achieve a variation
in spatial resolution around the ROI, some of the detector heads, for example detector
heads 1210a, 1210c, and 12104, are moveable circumferentially on gantry 1209 as indicated
by arrows 1212a, 1206c, and 1212e. As a result, in the embodiments of both Figs. 12A
and 12B,there can be more detectors in some areas, and in other areas, there can be
fewer detectors. Optionally, detector heads can be concentrated in the wide areas
to provide enhanced resolution in those areas. Optionally or alternatively, different
detector head qualities are used in different areas, for example, to support non-uniform
data collection protocols.
[0304] A possible benefit of the translational embodiment of Fig. 12A is that it may be
easier to implement. Arcuate motion, or translation over a range of ± about 15 to
30 degrees, for example, ±20 degrees from a central position can give good results.
[0305] Another way to enhance resolution around an ROI with a reduced number of detector
heads is to provide gantry rotation for PET operation as in SPECT operation. This
concept is illustrated in Fig. 13. In a non-rotating system, reducing the bore size
from the conventional 90cm to 30cm for a small ROI for a particularly configured detector
head results in a larger angle of acceptance α2 as compared to α1, but may decrease
the angular resolution, e.g., as previously noted. However, if the gantry is continuously
rotated, or continuously rotated during imaging of successive axial slices, e.g.,
with the same number of detector heads, and a bore size of 30 cm, the angle of acceptance
α3 can be made smaller, for example, smaller than even α1, and the lost angular resolution
can be recovered. Optionally, the speed of rotation is selected according to the desired
acceptance angle. In an exemplary embodiment of the invention, with a rotating gantry,
the detector array can be configured with a number of detector heads arranged over
less than the full 360 degrees around the ROI, for example, over between 180 and 320
degrees. In a static system, that would result in a potentially large gap in coverage.
Rotation assures that emission events from all parts of the ROI will be detected as
the detector array rotates, even though generation of the image data may require more
time.
[0306] A rotating gantry can also be provided in embodiments in which the detector heads
are mounted on axially spaced gantry rings and/or translatable on the gantry, yielding
the benefits of both a wide range of bore configuration adjustability and increased
angular resolution with fewer detector heads. Optionally, the two gantry rings can
be arranged to rotate at different speeds.
[0307] Fig. 14 illustrates a potential benefit of varying the detector geometry during a
full-body scan. For a normal patient 1402, a small bore is used for the slices in
the regions 1402a and 1402b of the lower legs, neck, and head, and a larger bore size
for the region 1404c of the upper legs and the torso. In contrast, for an obese patient
1404, a larger number, for example, four bore sizes gives better results. Thus, for
regions 1404a and 1404e covering the lower legs, the head and the neck, a first bore
size is used. For regions 1404b and 1404c covering the upper legs and the upper torso,
a second larger bore size is used. For region 1404d covering the lower torso and the
chest, a third bore size, even larger than those for the other regions is used.
[0308] A further option is to vary the bore size on-the-fly as the scan proceeds, resulting
in a bore size that dynamically follows the contour of the patient's body.
[0309] It should also be noted that for an ROI that is relatively small, decreasing the
bore size can be facilitated by making the patient carrier transversely adjustable
or with a part that is narrower than the overall width.
Exemplary Method of Use:
[0310] While it is believed that the method of use of the various embodiments described
above should be apparent to those skilled in the art from the foregoing description,
this may be summarized in conjunction with the flow diagram of Fig. 15 and 16. For
purposes of discussion, it is assumed that a PET procedure or a SPECT procedure is
to be performed either in a single or dual mode system, but it should be understood
that the discussion is also applicable to simultaneous performance of PET and SPECT
procedures.
[0311] As shown, at 1502, suitable preparation of the patient, including injection of the
radioactive tracer is optionally undertaken. At 1504, optionally while (and/or before,
and/or after) the tracer is circulating through the bloodstream to the ROI, the size
and shape of the ROI is determined, for example by a conventional transmission CT.
Optionally, the CT may be performed using a CT capability (e.g., using an x-ray or
radiation source) included in the N-M tomography system itself, or by use of a separate
CT system.
[0312] At 1506, the bore geometry and if necessary, the configuration of the collimator
septa are adjusted to accommodate the size, shape, and location of the ROI and the
desired spatial resolution according to the nature of the procedure being performed.
Depending on the required size of the bore, the detectors are extended as needed.
If the detectors cannot be extended sufficiently to provide as small enough bore,
only some of the detectors are extended. Alternatively, according to the features
of the particular system, the detectors are rotated and/or tilted to the required
angular orientation, and optionally then the detectors are extended.
[0313] At 1508, after sufficient time (and/or during this time) for the tracer to travel
through the patient's bloodstream to the ROI, the scan is performed, and at 1510,
the image reconstruction is performed.
[0314] Fig. 16 illustrates a more complex scan procedure, again applicable in general to
a PET or a SPECT procedure, or simultaneous performance of both PET and SPECT procedures.
Here, 1602 and 1604 are the same as 1502 and 1504, but at 1606, a scan regimen is
programmed for in-scan detector and collimator geometry variation. This may include
one or more of the following features:
- a) Preliminary bore size adjustment;
- b) Continuous gantry rotation during the scan or at each axial slice;
- c) Gantry rotation speed adjustment;
- d) Continuous bore size adjustment (both extension and angular orientation of the
detector heads) during the scan at particular axial positions, or continuously over
the entire scan;
- e) Variable positioning of the detector heads on the gantry (initially or over the
course of the scan);
- f) Variable collimator configuration initially and/or over the course of the scan.
[0315] At 1608, after sufficient time for the tracer to travel through the patient's bloodstream
to the ROI, the scan is performed according to the programmed regimen, and at 1610,
the image reconstruction is performed.
Detector Unit Arrangements and Configurations:
[0316] Referring again to Fig. 2, it will be recalled that a conventional SPECT detector
unit is comprised of an emission detector element 32 in the form of a scintillator
that provides an optical (or electrical) light pulse in response to impingement of
a gamma-ray photon, an array of photomultiplier tubes (PMT) 34 that convert the optical
light pulses into electrical signals from which the images are reconstructed and a
collimator arrangement 42 with openings aligned with the PMTs to provide a narrow
acceptance angle for each PMT, i.e., to ensure that photons striking the detectors
do so at a relatively narrow range of angles. Alternatively, the scintillators and
the PMT can be replaced by a direct conversion semiconductor detector, for example,
a SiPM as described herein.
[0317] Conventional PET detector units can be similarly configured, but do not include a
collimator array because incidence angle information is extracted based on coincident
detection of two photons emitted by a single radioactive decay
Scintillation detector elements are typically formed as unitary structures, and such
structures are also employed in some embodiments of the invention. Alternatively,
the detector elements according to some embodiments are pixilated, e.g., formed of
an array of discrete small detector pixels. This can be advantageous particularly
for PET imaging, in that it enables identifying the orientation from which the photon
has been emitted at a much finer precision, for example, along a line with a width
of about 4-6 mm, between two locations of coincident detections taking into account
the distance of positron travel before annihilation and the pixel width.
[0318] In an exemplary embodiment of the invention, dual use (PET and SPECT) detector systems
are provided.
[0319] In an exemplary embodiment of the invention, pixilation may in some cases facilitate
optionally providing time-of-flight analysis circuitry for PET operation to determine
where along the estimated emission line the positron was emitted, for example at a
longitudinal resolution of, for example, about 1-5 cm, for example 2-3 cm (e.g., by
measuring such time per pixel or group of pixels).
[0320] Optionally, the electronic circuitry connected to some or all of the detector units
can also provide photon energy characterization (e.g. energy level) within the entire
SPECT and PET range of about 40 KeV to 511KeV, and detection of count rate in case
of high flux of photons, for example when a high intensity radiation source is activated
such as X-ray source, and/or detection time resolution sufficient for coincidence
and time of flight detection.
[0321] Optionally, the signal processing electronics can also include multiple separate
channels that allow independent amplification and front-end processing for each detector
or small group of detectors and/or a small number of pixels (e.g., between 10 and
1000, for example 100), such that any malfunction of one or more pixels or detectors
and any blinding of one or more pixels or detectors by a "hot spot" (high intensity
source) do not prevent other detectors from properly functioning and detect photons
emitted from other regions. One suitable way to achieve this is shown in commonly
assigned
U.S. patent 8,445,851 commonly owned herewith, the content of which is hereby incorporated herein in its
entirety as if fully set forth. The detector pixels can be arranged in various configurations
according to embodiments of the invention. In an example, the detector can be configured
as a symmetrical matrix of 8x8 pixels, 10x10 pixels, 12x12 pixels, 16x16 pixels 20x20
pixels, 32x32 pixels, or larger or smaller or intermediate sized matrices. Alternatively,
the pixels may be arranged in asymmetric configurations, for example 16x32 pixels,
16x64 pixels, 8x16 pixels, and other larger, smaller or intermediate sized configurations.
[0322] In a non-limiting example, detector pixels have dimensions in the range of about
0.1-20mm, for example, about 0.2-15mm, for example 0.5-10mm, for example 1-5mm, for
example 1-2mm or 2-3mm or 2-4mm.
[0323] While in an exemplary embodiment of the invention the pixels are symmetric (e.g.,
square), this need not be the case, for example, the pixels may be elongate in a certain
direction, for example, having a factor of between 1.1 and 4 or more between two orthogonal
dimensions thereof.
[0324] In another non-limiting example, the pixel pitch (i.e., spacing between pixels),
is symmetrical in two directions, for example, about 2.5mm or about 1.25mm or about
1mm, or about 2mm or about 3mm, or larger, or smaller or intermediate values. In another
example, the pixel dimension in one direction is different than the dimension in another
direction, for example 2x3mm, 1.5x2.5mm, 2x2.5mm, etc.
[0325] In some exemplary embodiments, the detectors have dimensions in the range of about
1cm to about 15cm, for example in the range of 2 to 8cm, for example in the range
of 3 to 5 cm, for example 4cm.
Exemplary reconstruction variations
[0326] In some exemplary embodiments of the present invention photons are detected by solid
state detectors and electronic circuitry that are configured for acquisition of single
photons of typical SPECT energy levels, and/or single photons of high energy such
as 511KeV, and/or coincidence detection of pairs of photon received as a result of
a single positron emission from the radiopharmaceutical. In exemplary embodiments
of the invention detector modules are capable of detecting more than one of these
modes, for example detecting a wide range of energies single photons such as from
40KeV to 511KeV. In other exemplary embodiments of the invention detector modules
are capable of detecting 51 IKeV photons both as single events (if coincidence of
a pair of photons was not detected) and as coincidence photons detection.
[0327] In an exemplary embodiment of the present invention a collimator is used on the detector
which provides wide collection angle of 511KeV, but with some preferred orientation
of detection (for example, about 20%, or 30%, or 50%, or 70%, or 100% or 150% or 200%
or 300% higher probability in a certain direction (e.g., having an angular aperture
of between 0.001 and 10 degrees in a largest dimension) compared with most other directions,
for example about 100% higher, which is about twice the probability, in a main direction).
[0328] In an example, information from photons that are detected as part of a coincidence
is processed by the reconstruction algorithm as being probably received from a location
along a line of sight between the two detection locations, and information from photons
that were detected only in one location with no detection of the coincidence can be
either ignored or being processed in a SPECT-like probability analysis based on the
detection probability function ("functional", detection probability map) which depends
on the collimator properties and its preferred orientation.
[0329] In an example, the reconstruction algorithm combines information from both SPECT-like
analysis and coincidence-like analysis. In an example, the analysis based on individually
detected photons (as a single detected photon, and/or as each of a pair of coincidence
photons) is used to form an interim information, for example interim reconstruction
of the radiopharmaceutical distribution in 3D volume, and that interim information
is used as a prior information to a further 3D image reconstruction based on coincidence
analysis. In this approach, for example, analysis based on one approach is either
fully integrated with, and/or iteratively integrated with, and/or used as a prior
info for, analysis based on the other approach. In an example, PET-like analysis serves
as prior knowledge for SPECT-like analysis. In an example, SPECT-like analysis serves
as prior knowledge for PET-like analysis. In an example, the SPECT-like analysis and
the PET-like analysis are iteratively performed, either one providing for preprocessing
or prior knowledge for the other, or one serves for post-filtering of the other, or
algorithms merged with one-another, or any combination thereof.
[0330] In an exemplary embodiment of the invention, when reconstructing data from multiple
energies, a priori probability of correlation between two energies is optionally used.
For example, for a given body structure, the apriori probability of a SPECT event
(or event at one energy) may be different from the a priori probability of a SPECT
or PET event at a different part of the structure and/or at the same part. Optionally,
a previous image is used as an input to indicate the differences in radiopharmaceutical
distributions at the different detected energies. In one example, heart muscle is
detected using one energy and a diseased location in the heart is detected using another
energy. Reconstruction of the shape and/or location of the heart using the first energy
may be used to limit (e.g., anchor) the other energy to fit within the boundaries
of the heart as reconstructed by the first energy or as matching a model sized and
shaped using the first energy.
[0331] In an exemplary embodiment of the present invention the reconstruction algorithm
is adaptive to take into account variable location of the detectors. Unlike conventional
PET, where the algorithm assumes that detectors positions and orientation is known
and fixed in advance, and in particular the
relative location and orientation of the detectors is known (one detectors relative to the
other detectors), in an example of the present invention the algorithm forms probability
distribution maps that factor the de-facto location of the detectors during the acquisition,
as customized per patient and/or instant of time.
[0332] In an example, the distance between detectors and the body, the location of the detectors
in space and the relative position of the detectors (one relative to others) varies
from one detector to another and from one patient to another. Moreover, in an example,
the algorithm is configured differently than conventional PET-reconstruction algorithms
to form probability maps for photons to be detected by a detector, to be calculated
based on the location and orientation of the detector during the acquisition. The
probability to obtain coincidence detection changes as the detectors move and are
positioned closer to the body, as the line of sight between any two detectors becomes
different than that which was prefixed in conventional ring-based detectors.
[0333] In an example, a 3D image reconstruction algorithm calculates a probability function
("the functional") of a radiopharmaceutical for a voxel (a small volume in a certain
3D location in space) to emit a positron that converts to 2 photons (following annihilation
of the positron) that would be detected as a coincidence event by a pair of pixels
(one from each of two opposing detectors), taking into account the position of the
detectors and their orientation as a result of the detector motion in-out towards
the body. In an example, the probability of detection as a single photon event is
calculated too, taking into account, for example, the position of the detectors as
a result of the detector motion in-out towards the body and/or collimator or detector
design. In an example, the orientation of the detectors is also being used as part
of the calculation of the probability function.
[0334] In an example, the detectors also move laterally, for example by motion of the gantry
and/or linear motion and/or rotation thereof, and the reconstruction algorithm forms
the probability functions taking into account the gantry lateral motion and its effect
on the position and orientation of the detectors. In some examples, such motion is
done before photon acquisition begins, and the algorithm accounts for it. In another
example, such motion occurs during photon acquisition process, and the algorithm accounts
for it by having the probability function calculated to take into account the dynamic
changes due to the relative motion during the scan.
[0335] In an example, the detectors rotate around one or more local axes, for example by
rotating around an axis of rotation per detector structure (or per group of detectors),
for example, independently rotating detectors (or groups) around an axis which is
more-or-less parallel to the main axis of the patient body. In this example, the reconstruction
algorithm forms the probability functions taking into account the detector rotation.
In some examples, such rotation is done before photon acquisition begins, and the
algorithm accounts for it. In another example, such rotation occurs during photon
acquisition process, and the algorithm accounts for it by having the probability function
calculated to take into account the dynamic changes due to the orientation changes
during the scan.
[0336] In an example, a combination of the some or all of above components of the reconstruction
algorithm is used to enable adaptation of the reconstruction algorithm and use of
the probability functions taking into account the ability of the detectors to move
before and/or during the scan. For example, the adaptation is provided using a sensitivity
or energy correction and/or by modifying the model of the detectors used in reconstruction.
As noted herein, in some cases planning or RT acquisition is modified, for example,
to ensure a sufficient photon count from a desired location, to ensure a desired quality,
to avoid data collection from undesired regions and/or to ensure stability of reconstruction.
[0337] In another example of the present invention the system is capable of simultaneously
acquire high energy (e.g. PET, 511 KeV) and lower energy (SPECT, X-Ray) photons. In
an example, such simultaneous acquisition of photons from multiple energy levels allows
simultaneous image acquisition of multiple radiopharmaceuticals. For example, simultaneous
imaging of radiation from PET isotope (e.g. radiopharmaceutical based on one or more
of F-18, C-ll, N-13, O-15, Rb-82, Cu-62, Ga-68, Iodine), and from a SPECT isotope
(e.g. radiopharmaceutical based on one or more of Tc
99m, T1
201, I
123, In
111). In an example, the acquisition of photons emitted by two or more radiopharmaceuticals
is simultaneous, and the image reconstruction algorithm generates 3D images of the
distribution of two or more radiopharmaceuticals within the ROI, thus avoids problems
associated with registration of images from different sources that are acquired by
different systems and/or at different time.
[0338] While the term PET has been used for convenience, other coincidence detection methods
may be used. Similarly, other single photon detection methods than SPECT may be used.
[0339] Such a system as described herein may be selectively operated, for example, in a
single mode (coincidence or non-coincidence) and/or in a dual mode.
[0340] In an exemplary embodiment of the invention, for coincidence detection, time stamps
per photon are obtained at about microseconds or sub-microsecond resolution, and time-of-flight
processing is optionally obtained if time-stamp is obtained at sub-nanosecond resolution.
In these cases, a processing means, such as a central CPU (in this or other embodiments)
can identify the matching photons and analyze the coincidence emission line, and if
available also the estimated position along the line based on time-of-flight calculation.
Collimator Arrangements and Configurations:
[0341] Figs. 17-22D illustrate exemplary collimator configurations, including, for example
designs that are adjustable to provide high and low spatial resolution. This capability
may be advantageous for SPECT imaging using N-M tomography systems providing adjustable
bore geometry as discussed below, and also to permit selectably using the same detector
units for PET or SPECT imaging, or for both PET and SPECT imaging simultaneously.
In some embodiments, no physical adjustment is applied, for example, using software
correction to adapt the received signal for different desired collimation conditions.
[0342] Referring first to Fig. 17, there is shown a representation of an exemplary basic
adjustable collimator designated 1700. For reference purposes, the Z-direction is
taken as perpendicular to the plane of the sensor element and the Y-direction is the
direction parallel to the machine or patient axis, The X-direction is orthogonal to
the Y and Z axes, and with the Y-axis defines a plane parallel to the plane of the
sensor element. It is note that non-rectangular collimators may be used, for example,
hexagonal.
[0343] As illustrated in Fig. 17, collimator 1700, is formed of two orthogonal sets of septa
1702 and 1704. Septa 1702 lie in X-Z planes (spaced in the Y-direction). Septa 1704
lie in Y-Z planes (spaced in the X-direction). Septa 1702 are formed with slits 1706
at spaced intervals in the X direction. Septa 1704 are formed with slits 1708 at spaced
intervals in the Y direction. The spacing between slits 1706 corresponds to the X-direction
spacing (or multiples thereof) of septa 1704, while the spacing between slits 1708
corresponds to the X-direction spacing (or multiples thereof) of septa 1702.
[0344] Septa 1702 and 1704 fit together as shown to form an "egg-crate" array of collimator
cells 1710 that can easily be assembled. As illustrated, the spacing of septa 1702
(in the Y direction) and 1704 (in the X direction) are the same so that cells 1710
are square. However, it should be understood that the septa spacing can be different
in the X and Y directions so that cells 1710 are rectangular. Another variation is
to make the slit spacing and/or the spacing of septa 1704 non-uniform allowing different
size cells at different locations in the collimator. These variations are simple to
achieve with the illustrated design. A plastic frame (not shown, optionally positioned
between the collimator and the ROI) is optionally used to press the septa together
and against the detector, for example, using one or more screws to provide the pressure.
Other attachment mechanism may be used as well, for example connecters which interconnect
the speta and/or attach a flange at the end of one or more septa to the detector.
[0345] 18A-22D illustrate exemplary (but non-limiting) ways to vary the resolution of collimators
according to some embodiments of the invention. Before proceeding to a description
of some of these embodiments, the following points should be noted:
- 1. Fig. 18A shows a fragment 1800 of a collimator formed by spaced Y-Z plane septa
1802 and X-Z plane septa 1804. These define collimator cells 1806 (four of which are
shown) that provide photon travel paths to the detector element. For simplicity, Figs.
18A-22D show only part of a single row of the collimator. The full collimator generally
includes multiple (e.g., 4, 10, 20, 30 or intermediate or greater numbers) parallel
rows like 1800. Also, while only four cells 1806 are shown, the actual number of cells
(as well as the number of parallel rows 1800) will depend on the overall dimensions
of the emission detector element, and the pitch of the septa (e.g., the spacing between
septa or septa centers).
- 2. In some embodiments, the septa pitch in the Y-direction is the same as that in
the X-direction whereby the individual collimator cells 1806 are square. Alternatively,
the septa pitch in the Y-direction is different from that in the X-direction whereby
the individual collimator cells 1806 are rectangular. Alternatively or additionally
the pitch can be variable in the X and/or Y directions. In some embodiments the septa
are not straight. For example, the arrangement may be of radially (e.g., extending
from one or more points) arranged fixed septa and circular speta mounted thereon.
Optionally or alternatively, one or more septa may be inclined away from a perpendicular
to the detector.
- 3. In some embodiments, in addition to or instead of different pitch in the X and/or
Y directions, and/or variable pitch in the X and Y directions, the septa thickness
may be different in the X and/or Y directions. As an additional or alternative option,
the septa thickness may be variable in one or both the X and Y directions. As another
alternative or additional option, the septa length may be different in the X and Y
directions. As yet another alternative or additional option, the septa length may
be variable in one or both the X and Y directions. The variation within a detector
collimator for one or more of these parameters may be, for example, within a factor
of 1.1, 2, 3, 4, or intermediate or greater factors. Thus, some embodiments provide
non-uniformly sized and/or shaped collimator cells.
- 4. Collimator configurations and geometry variations as illustrated in Figs. 18A-22D
can be used with both pixilated and with non-pixilated detectors.
- 5. Conventional PET detectors do not include collimators. However, it has been found
that certain materials, for example, tungsten and the others mentioned above more
efficiently absorb photons having energy in the 40-250KeV range emitted by tracers
used for SPECT imaging than the high energy (511KeV) PET photons. Forming the collimator
septa of such materials helps make it possible to use detectors having collimators
as described herein for both PET and SPECT imaging with only slightly less efficiency
(i.e., sensitivity, e.g., 30%, 20%, 10% or intermediate or smaller reduction of sensitivity)
in the PET mode, but with wider effective viewing angle (i.e., angle of acceptance)
since the high energy photons are able to pass multiple septa, for example, 3, 4,
5 or 6 septa. Stated differently, despite the decrease in detection probability (resulting
from decreased sensitivity as the angle of acceptance increases), useful SPECT detectors
can still provide high effective sensitivity for PET detection.
- 6. In exemplary embodiments, blockage for PET energies is less than 80%, 60%, 50%,
20% or intermediate percentages depending, for example, on the acceptance angle, septa
design, and/or material as described herein. As a specific non-limiting example, the
collimator may be formed of tungsten septa about 0.2mm in thickness, and having 1.03mm
square cells, with a pitch of 1.23mm and height in the Z-direction of 14.5mm, and
overall horizontal septa length of 10.8mm.
- 7. Use of wide angle reconstruction techniques for SPECT imaging, for example, the
ML-EM algorithm, allows a single adjustable-geometry collimator to be used for SPECT
imaging for multiple distances between the detector and the target area, e.g., a range
factor of 1.5, 2, 3, 5 or intermediate or greater values. Suitable known reconstruction
techniques for PET imaging include without limitation, FBP (filtered back projections),
iterative algorithms with or without PSF (point spread function), and modeling. These
may also permit collimated detector units formed for example, of tungsten septa to
be used for PET imaging to detect photons over a range of detector angular extent
along the system axis, for example, e.g., a range factor of 1.5, 2, 3, 5 or intermediate
or greater factors.
- 8. It should further be noted that conventional SPECT algorithms assume a collection
angle and a detection probability map (resulting from the collimation and perhaps
other factors) that is part of the algorithm. In PET imaging, as the detectors are
exposed to the entire imaged volume, the coincidence line is used for the reconstruction
process. However, in the some of the exemplary embodiments, the variable septa geometry
affects PET reconstruction to some extent, in steps - sensitivity being changed (and
generally reduced with some steps) as the detection angle increases. In an exemplary
embodiment of the invention, the algorithms suitable for PET image reconstruction
advantageously take account of the septa configuration to attribute to photons coming
from a particular coincidence line a detection probability, such that the probability
is factored into the calculation: lower probability means that the source in that
direction is actually "hotter"/"brighter" than it looks. Therefore, the value attributed
to radiation received by a particular pixel is related to the counts received from
that direction divided by the probability of detection from that location. This concept
is valid for both SPECT and PET reconstruction because the probability of detection
varies in different angles related to the existence of the collimator and the septa
configuration, but also the fact that different angles of detection hit different
effective thickness of septa, the fact that collimation may be variable, and/or the
variable geometry of the detector heads as previously described.
[0346] Figs. 18A-18C illustrate an embodiment in which spatial resolution is enhanced by
lengthening the Y-Z plane septa 1802 and/or the X-Z septa 1804 thereby narrowing the
angle of photon acceptance.
[0347] In Fig. 18A, collimator fragment 1800 is shown in an un-elongated configuration which
provides a wide angle of acceptance for SPECT imaging, for example, ranging from about
1 and about 30 degrees, and advantageously, between about 5 and about 15 degrees Fig.
18B shows elongation of the collimator in the Z-direction to reduce the angle of acceptance
and thereby improve the spatial resolution. Fig. 18B shows extension of only the X-Z
plane septa 1804, while Fig. 18C shows elongation of both the X-Z septa and the Y-Z
septa. It should be understood that alternatively for the situation illustrated in
Fig. 18B, the Y-Z plane septa 1802 can be extended instead of X-Z plane septa 1804.
[0348] Extension and retraction of septa 1802 and 1804 may be achieved by any suitable and
desired mechanism. This is shown schematically as a coupling rod 1806 for septa 1804,
A small motor, for example a stepper motor or a multiple position relay (not shown)
attached to coupling rod 1808 provides for step-wise movement. Alternatively, continuous
adjustment can be provided, for example, by a suitable motor and position sensors.
The septa can also be extended and retracted manually.
[0349] Extending either septa 1802 or 1804 as described can be useful, for example to increase
resolution in a certain orientation - while possibly reducing sensitivity in that
direction. Retracting the septa does the opposite. In an exemplary embodiment of the
invention, tilting of speta (e.g., by moving a bore-side thereof while using the detector
side as a pivot) is used for when the detector is rotated out of plane during arrangements
as described above. A louver-like mechanism may be used.
[0350] In an exemplary embodiment of the invention, the reconstruction algorithm is adapted
for the collimator configuration, for example, based on a look-up table with different
parameters for different collimator configurations. Optionally, a sensor which reports
the collimator configuration or the command for collimator adjustment is used to calculate
such parameters and/or as input to a reconstruction algorithm. Which uses such measurement
to modify the reconstruction (e.g., sensitivity correction and desired count rate
for stability of reconstruction and/or image quality).
[0351] In an exemplary embodiment of the invention, planning of data acquisition takes into
account possible collimator variations, for example, by calculating desired configurations
and/or by comparing options with different collimator arrangements.
[0352] In an exemplary embodiment of the invention, when acquisition depends on pervious
acquisition, collimator and/or detector configuration are changed in a manner which
preferentially provides data (photon detections) from a desired location and/or time
and/or to preferentially block data from a certain location and/or time.
[0353] Figs. 19A and 19B illustrate an embodiment in which the pitch of the septa is varied.
Fig. 19A shows a collimator fragment 1900 comprised of X-Z plane septa 1902 and Y-Z
plane septa 1904 defining collimator cells 1906. Fig. 19A shows a configuration in
which the X and Y dimensions of the collimator elements are equal. Fig. 19B shows
X-Z plane septa 1902 shifted in the Y-direction by one-half the septa pitch. Alternatively,
Y-Z plane septa 1942 may be shifted (in the X-direction). In either case, the cross-sectional
area of the opening in collimator cells 1906 is reduced by for example, 25-75 percent,
for example, 50 percent thereby decreasing the acceptance angle and increasing the
spatial resolution.
[0354] In some embodiments, both (some or all thereof) the X-Z and Y-Z plane septa 1902
and 1904 can be shifted, thereby reducing the area of the collimator cell openings,
for example, by 75 percent, and further decreasing the acceptance angle and correspondingly
increasing the spatial resolution.
[0355] Moving septa 1902 and/or 1904 can be effected using an arrangement similar to that
described in connection with Figs. 18A-18C (but adapted to provide Y-direction movement)
or in any other suitable and desired manner.
[0356] Figs. 20A and 20B illustrate another embodiment in which the pitch of the septa is
varied. In Fig. 20A, a collimator fragment 2000, is formed by X-Z plane septa 2002
and Y-Z plane septa 2004 defining collimator cells 1906. As in Fig. 19A the septa
pitch is the same for septa 2002 and 2004.
[0357] Fig. 20 shows the upper ends of X-Z plane septa 2002 tilted in the Y-direction by
one-half the septa pitch. Alternatively, Y-Z plane septa 1942 may be shifted (in the
X-direction). In either case, the area of opening in collimator elements 1906 is reduced
by, for example, by 25-75 percent, for example, 50 percent thereby decreasing the
acceptance angle and increasing the spatial resolution.
[0358] The tilting may be achieved in generally the same manner as in the embodiments of
Figs. 18 and 19, except that the bottom ends 2008 of septa 2002 are pivotally mounted.
In some embodiments tilting provides a parallel collimation. In other embodiments,
it provides a fan-in collimation or a fan-out collimation. Different adjustment and
adjustment types may be provided for different detectors and/or for different parts
of a same detector, for example, during a same session or even simultaneously, for
example, responsive to ROI location, ROI type, time form injection of tracer and/or
arm and/or bore geometry.
[0359] Figs. 21A-21D illustrate resolution adjustment using an arrangement of layered or
vertically tandem collimator sub-units or parts, in accordance with some embodiments
of the invention. Figs. 21A and 21B are respectively an end elevation and a side perspective
view of a two part collimator fragment 2100 comprised of first sub-unit 2102 and a
second sub-unit 2104 located above sub-unit 2102. In this context, the word "above"
is to be understood as meaning closer to the detector element. Therefore, sub-unit
2102 is positioned closer to the source of radiation than sub-unit 2104.
[0360] Sub-unit 2102 is formed a first set of septa 2106 extending in an X-Z plane, and
a second set of septa 2108 extending in a Y-Z plane. Sub-unit 2104, in contrast, is
formed only of one set of septa 2110 extending in the X-Z plane. Alternatively, sub-unit
2104 can be formed of two sets of septa like sub-unit 2102.
[0361] Also, while sub-unit 2104 is above sub-unit 2102, alternatively, the sub-units can
be reversed so that sub-unit 2102 is above sub-unit 2104.
[0362] Fig. 21B shows two ways the collimator cells of collimator 2100 may be decreased
in size to provide a smaller acceptance angle, and therefore higher resolution for
SPECT imaging: septa 2108 can be moved in the X-direction and septa 2110 can be moved
in the Y-direction. If only septa 2108 are moved, the area of the cell openings is
decreased by up to 50 percent. If both sets of septa 2108 and 2110 are moved, cell
opening area can be decreased by up to an additional 25 percent.
[0363] Fig. 21C illustrates an alternative collimator configuration 2112 comprised of an
upper sub-unit 2114 formed by X-Z plane septa 2118 and a lower sub-unit 2116 formed
by X-Z plane septa 2120 and Y-Z plane septa 2122. In this embodiment, sub-unit 2114
includes a second set of X-Z plane septa 2124 positioned between septa 2110, and sub-unit
2102 includes a second set of Y-Z plane septa, one of which is shown at 2126 positioned
between septa 2122. In this configuration, only intermediate septa 2114 and 2126 are
moveable.
[0364] Fig. 21D shows a further alternative embodiment 2128 in which the septa are configured
as in the embodiment of Fig. 21B except that the septa 2122 and 2130 are tiltable
rather than slidable in the Y and X directions, respectively.
[0365] An un-illustrated variation of collimator 2112 of Fig. 21C has three sub-units or
parts in vertically tandem relationship. The three parts may be of equal length in
the Z-direction, or any other desired proportion, for example, 1:2:1 (i.e., so that
middle part is twice as long as the top and bottom parts and thereby provides one-half
the length of the collimator. Other proportions are also possible.
[0366] Optionally, collimator resolution in this embodiment is increased by moving the Y-Z
plane septa forming the middle part in the X and/or Y directions as in the embodiment
of Fig. 21C. Optionally or additionally, septa forming the top and bottom parts are
moved.
[0367] Another un-illustrated three-part collimator is similar to collimator 2128 of Fig.
22D, in which resolution is increased by tilting the septa of the central part.
[0368] The three layer configurations may be desirable in that they may provide a more symmetrical
high resolution pattern in the X and Y directions.
[0369] Figs. 22A-22D illustrate embodiments in which the opening area of the collimator
cells is changed by shutter-like mechanisms. In Figs. 22A and 22B, collimator fragment
2200 is formed by X-Z plane septa 2202 and Y-Z plane septa 2204 that define collimator
cells 2206. At the upper ends of cells 2006 are pairs of cooperating triangular shutter
leaves 2208 and 2210. Shutter leaves 2208 may be fixed in place while leaves 2210
are slidable in the X direction, for example, by an actuator rod 2212. In the open
position illustrated in Fig. 22A leaves 2210 substantially overlie leaves 2208. In
the closed position illustrated in Fig. 22B leaves 2210 have been shifted to partially
close the tops of cells 2006.
[0370] Fig. 22C illustrates an embodiment in which the tops of the collimator cells 2206
are closed by rotating flaps or leaves 2214 on a mechanism shown schematically as
an actuator rod 2216.
[0371] Fig. 22D illustrates an embodiment in which the tops of the collimator cells 2206
are closed by an iris-like shutter 2218. This embodiment may be advantageous in that
it allows achievement of very small area openings for the collimator cells. Actuation
of shutter 2218 may be by a convention rotating mechanism, as in a photographic camera,
or in any other suitable and desired way.
[0372] The dimensions of the collimators illustrated in Figs. 18A-22D may be varied over
ranges, for example, as indicated in the non-limiting examples given below.
- 1. The pitch of the collimator septa may be in the range of about 1mm to about 3mm.
Larger or smaller pitch values are also possible.
- 2. Typical septa thickness may be in the range of about 0.2mm to about 0.3mm. Again,
larger or smaller thicknesses are also possible.
- 3. The height of the septa (in the Z-direction) may be in the range of about 13mm
to about 25mm, or larger or smaller values.
- 4. The length of the septa (in the X and Y directions) may range from about 8mm to
about 20mm or larger or smaller values.
Exemplary Relationships Between Collimator and Pixilated Detector Configurations:
[0373] The following discussion describes some embodiments as non-limiting examples of collimator
configurations in relation to pixilated detectors, using the above collimator designs
or using other collimators, such as machined slabs.
[0374] In a first embodiment, the collimator septa are aligned with the pixel-pitch of the
detector, i.e., the septa are positioned at the borders of each pixel. In such a configuration,
the collimator cells are aligned with the detector pixels with one pixel per collimator
cell.
[0375] In another embodiment, the septal pitch in one direction, (e.g., the X direction
as defined in connection with Figs. 17-18), or the other direction (or both) is greater
than the pixel pitch, for example, in an integer ratio, of 1:2 or 1:3, or 1:4, or
1:5, or greater. In such an arrangement, if the septal pitch matches the pixel pitch
in one direction, and is two times the pixel pitch in the other direction, there will
be two pixels within each collimator cell. If the septal pitch is twice the pixel
pitch in both directions, there will be four pixels in each collimator cell. This
configuration may allow the generation of multiple different views within the same
collimator cell. In other embodiments the septa are not aligned with some or all pixel
boundaries.
[0376] In a further embodiment, all the septa in one or both the X-Z and Y-Z planes (also
as previously defined may be oriented so that they are not parallel to each other.
This configuration forms a collimation structure in which multiple pixels have different
views, passing through the same cell. In one example, a multiple pinhole structure
is provided. In one example, multiple apertures are provided. In another example,
the multiple apertures are arranged to form coded aperture collimation structure,
for example of a type known in the art.
[0377] It should be noted that coded aperture techniques are known to those skilled in the
art for use in gamma ray imaging, and will not be described here in the interest of
brevity.
[0378] In another embodiment, the septa pitch is smaller than the pixel pitch, for example,
according to an integer ratio, of 1:2, or 1:3, or 1:4, or 1:5 or greater. This may
be achieved by positioning one or more additional septa at the middle of a pixel to
provide, multiple collimator cells within each pixel. With this configuration it is
possible to obtain a particular viewing angle (collection angle) with shorter collimator
septa. For example, if for a certain desired collection angle one would use a pixel
pitch of 2.5mm and a single collimator cell per pixel with septa length of about 20mm,
similar performance can be obtained by providing two collimator cells per pixel with
a septa length of 10mm. Shorter septa may be advantageous since they may permit having
a smaller detector head with better maneuverability.
[0379] In another embodiment, the collimator cell pitch is different in the X and Y directions,
and also different than the pixel pitch. For example, the collimator septa may be
pitched at 2mm in one direction and at 3 mm in the orthogonal direction. In general,
the septa spacing may be larger or smaller than the pixel spacing and also different
in the X and Y directions/pitch. For example, N collimator septa may be evenly spread
over K pixels in the X direction, and M collimator septa may be evenly spread over
L pixels in the Y direction.
[0380] In a further exemplary embodiment of the invention the length of the collimator septa
is different in the X and Y directions. For example, in the case of pixel pitch of
about 2.5mm, the X-Z plane septa can be about 18mm long and the Y-Z plane septa can
be about 24mm long. With this exemplary configuration it is possible for a pixilated
detector having square pixels to provide different view angles and collection angles
in each direction and to allow sensitivity and resolution of reconstruction to be
optimized where the camera scanning is very asymmetric in its nature.
[0381] For example: a camera scan and reconstruction may form many X-Z "slices" along the
Y axis which is parallel to patient body main axis and the Y resolution of the detector
is very influential on the reconstruction resolution in the Y axis. This is different
than the X-Z resolution, as the depth dimension is obtained by different views and
rotations within the X-Z plane, where the X resolution of detector is just one factor
and the distance, rotations, translations and reconstruction algorithm determine the
resulting resolution. In these cases it may well be that an improved result can be
obtained with a collection angle which is different in X axis than in Y axis. This
is optionally achieved with this unique approach of different septa length in the
X-Z and Y-Z planes.
Example
[0382] Figs. 23A-23C illustrate qualitatively the result of a simulation study performed
on an exemplary collimated detector as described herein formed of 0.2 mm tungsten
septa. In Fig. 23A, the horizontal axis represents ±θ, the angle between an approach
path 2302 from an emission event 2303 off the centerline 2304 of a collimator cell
2306 (see Fig. 23B), two curves are shown: curve 2308 for a typical SPECT isotope,
and 2310 for a typical PET isotope. As may be seen, SPECT performance is very directional,
but for small values of θ, PET performance is comparable.
[0383] However, for PET isotopes, off-axis detection is reduced, but not by so much as to
prevent use of collimated detectors according to some embodiments hereof for selectable
or simultaneous SPECT and PET imaging.
[0384] Fig. 23C provides an understanding of the saw-tooth shape of the off axis detection
probability for PET imaging. Here, it may be seen that the path 2310 for an emission
event at some off-axis position 2308 must pass through two septa 2312 and 2314 to
impinge on the center of a detector pixel 2316, and suffers additional attenuation.
[0385] Moreover, having the reconstruction algorithm compensate for the off-axis attenuation
(e.g., with angle dependent sensitivity weighting, e.g., calculated and/or measured
during calibration) can potentially improve PET performance significantly.
[0386] As used herein the term "about" refers to ± 10 %.
[0387] The terms "comprises", "comprising", "includes", "including", "having" and their
conjugates mean "including but not limited to".
[0388] The term "consisting of' means "including and limited to".
[0389] The term "consisting essentially of' means that the composition, method or structure
may include additional ingredients, steps and/or parts, but only if the additional
ingredients, steps and/or parts do not materially alter the basic and novel characteristics
of the claimed composition, method or structure.
[0390] As used herein, the singular form "a", "an" and "the" include plural references unless
the context clearly dictates otherwise. For example, the term "a compound" or "at
least one compound" may include a plurality of compounds, including mixtures thereof.
[0391] Throughout this application, various embodiments of this invention may be presented
in a range format. It should be understood that the description in range format is
merely for convenience and brevity and should not be construed as an inflexible limitation
on the scope of the invention. Accordingly, the description of a range should be considered
to have specifically disclosed all the possible subranges as well as individual numerical
values within that range. For example, description of a range such as from 1 to 6
should be considered to have specifically disclosed subranges such as from 1 to 3,
from 1 to 4, from 1 to 5, from 2 to 4, from 2 to 6, from 3 to 6 etc., as well as individual
numbers within that range, for example, 1, 2, 3, 4, 5, and 6. This applies regardless
of the breadth of the range.
[0392] Whenever a numerical range is indicated herein, it is meant to include any cited
numeral (fractional or integral) within the indicated range. The phrases "ranging/ranges
between" a first indicate number and a second indicate number and "ranging/ranges
from" a first indicate number "to" a second indicate number are used herein interchangeably
and are meant to include the first and second indicated numbers and all the fractional
and integral numerals therebetween.
[0393] It is appreciated that certain features of the invention, which are, for clarity,
described in the context of separate embodiments, may also be provided in combination
in a single embodiment. Conversely, various features of the invention, which are,
for brevity, described in the context of a single embodiment, may also be provided
separately or in any suitable subcombination or as suitable in any other described
embodiment of the invention. Certain features described in the context of various
embodiments are not to be considered essential features of those embodiments, unless
the embodiment is inoperative without those elements.
[0394] Although the invention has been described in conjunction with specific embodiments
thereof, it is evident that many alternatives, modifications, and variations will
be apparent to those skilled in the art. Accordingly, it is intended to embrace all
such alternatives, modifications and variations that fall within the spirit and broad
scope of the appended claims.
[0395] All publications, patents and patent applications mentioned in this specification
are herein incorporated in their entirety by reference into the specification, to
the same extent as if each individual publication, patent or patent application was
specifically and individually indicated to be incorporated herein by reference. In
addition, citation or identification of any reference in this application shall not
be construed as an admission that such reference is available as prior art to the
present invention. To the extent that section headings are used, they should not be
construed as necessarily limiting.