Field of the Invention
[0001] The invention relates to a method for direct conversion of plastic materials into
methane and/or liquid fuels and the use of ruthenium-modified zeolite to catalyse
this conversion.
Background of the Invention
[0002] Plastics have become useful and versatile materials with a wide range of applications.
More and more new polymeric materials are developed to meet increasing demands. Plastics
and other polymers are in theory recyclable, but after a relatively short functional
life, are destined to arrive as a significant component of waste. Most of these plastics
and other polymers disposed of in landfills are chemically stable and degrade minimally.
This is an increasing problem of plastic pollution in the environment, while the demand
for plastics keeps on increasing in most consumer products.
[0003] There are several well-known technologies for plastic polymer oxidation and degradation
such as thermal decomposition, incineration, photochemical and electrochemical oxidation.
Another solution to reduce environmental and economic impacts correlated to the accumulation
of these plastic polymers is closed-loop recycling wherein plastic material is mechanically
reprocessed to manufacture new products. For example, polyethylene terephthalate (PET),
polyethylene (PE) or polypropylene (PP) wastes are subjected to successive treatments
leading to recycled PET, PE or PP which are collected, sorted, pressed into bales,
crushed, washed, chopped into flakes, melted and extruded in pellets and offered for
sale. Then, these recycled PET, PE or PP may be used to create textile fibers, plastic
tubes for the construction industry or plastic films, plastic sheets, or new packaging
such as flasks or blister packs, etc.
[0004] However, these plastic recycling processes require an efficient upstream sorting
process and use huge amounts of energy, particularly during the extruding step. The
equipment used is also expensive, leading to high prices, which may be non-competitive
compared to virgin plastic. Moreover, the recycled plastic gradually loses its interesting
properties (rigidity, thermal resistance, etc.) due to the recycling process, and
become less interesting compared to virgin plastics.
[0005] Therefore, there exists a need for an effective, less expensive, robust and practical
technology for degrading plastic waste and simultaneously producing value-added products.
Summary of the Invention
[0006] An aspect of the present invention provides a method for converting one or more plastic
polymers into methane and/or liquid fuels comprising
- a) contacting one or more plastic polymers with a ruthenium-modified zeolite catalyst
in water to provide a reaction mixture;
- b) placing the reaction mixture under the pressure of 40 to 200 bars of H2 at 140 to 300 °C;
- c) cooling the reaction mixture to 20 to 25 °C;
- d) recovering methane from the reaction mixture; and/or
- e) recovering liquid fuels from the reaction mixture.
[0007] Another aspect of the present invention provides a method for preparing a ruthenium-modified
zeolite catalyst for direct conversion of one or more plastic polymers into methane
and/or liquid fuel comprising
- a) providing a zeolite
- b) preparing a mixture of ruthenium (Ru) salt and the zeolite of step a) in a solvent;
- c) stirring the mixture of step b) at 20 to 100°C and cooling the mixture to 20 to
25°C;
- d) adding NaBH4(aq) in the mixture of step c);
- e) stirring the mixture of step d);
- f) recovering ruthenium-modified zeolite catalyst.
[0008] A further aspect of the present invention provides a ruthenium-modified catalyst
obtained by the method of the invention.
[0009] A further aspect of the present invention provides a use of the ruthenium-modified
zeolite catalyst obtained by the method of the invention for direct conversion of
one or more plastic polymers into methane and/or liquid fuel.
Brief description of figures
[0010]
Figure 1 illustrates the synthesis of solid phase Ru catalyst a) FAU-zeolite (type X); b)
Ion exchange of Na+ by Ru3+; c) Ru-FAU.
Figure 2 shows SEM images of the FAU-zeolite (top) and the Ru-FAU catalyst (bottom).
Figure 3 shows a1) SEM image of the FAU-zeolite support; a2) SEM image of the Ru-FAU catalyst;
b1) HRTEM image of the FAU-zeolite support; b2) HRTEM image of the Ru-FAU catalyst,
yellow circle: crystalline Ru nano-structures; c1) HAADF-STEM image of the Ru-FAU
catalyst; c2, c3, c4) STEM elemental mapping of the Ru-FAU catalyst: Ru, Si, Al, respectively.
Figure 4 shows a1) HAADF-STEM image of the Ru-FAU catalyst; a2) HAADF-STEM with integrated
elemental mapping image (Ru, Si, and Al; smoothed); b1, b2, b3) STEM elemental mapping
of Ru-FAU: Ru, Si and Al, respectively.
Figure 5 shows EDX of the Ru-FAU catalyst (Region 1).
Figure 6 shows EDX of the Ru-FAU catalyst (Region 2).
Figure 7 shows EDX of the Ru-FAU catalyst (Region 3).
Figure 8 shows BET isotherms (N2) of the FAU-zeolite and Ru-FAU materials; b) Powder XRDs of the FAU-zeolite and Ru-FAU
materials.
Detailed description of the Invention
[0011] All publications, patent applications, patents, and other references mentioned herein
are incorporated by reference in their entirety. The publications and applications
discussed herein are provided solely for their disclosure prior to the filing date
of the present application. Nothing herein is to be construed as an admission that
the present invention is not entitled to antedate such publication by virtue of prior
invention. In addition, the materials, methods, and examples are illustrative only
and are not intended to be limiting.
[0012] In the case of conflict, the present specification, including definitions, will control.
Unless defined otherwise, all technical and scientific terms used herein have the
same meaning as is commonly understood by one of skill in art to which the subject
matter herein belongs. As used herein, the following definitions are supplied in order
to facilitate the understanding of the present invention.
[0013] The term "comprise" is generally used in the sense of include, that is to say permitting
the presence of one or more features or components. In addition, as used in the specification
and claims, the language "comprising" can include analogous embodiments described
in terms of "consisting of' and/or "consisting essentially of".
[0014] As used in the specification and claims, the term "and/or" used in a phrase such
as "A and/or B" herein is intended to include "A and B", "A or B", "A", and "B".
[0015] As used in the specification and claims, the singular forms "a", "an" and "the" include
plural references unless the context clearly dictates otherwise.
[0016] The present invention reports a ruthenium-modified zeolite that efficiently transforms
plastic polymers to methane and/or liquid fuels, using water as the solvent. The catalyst
achieves high product selectivity and no significant ageing effect was observed after
multiple cycles. The resulting methane can directly be used as an energy carrier in
the form of H
2-enriched methane.
[0017] The method of the present invention for direct converting one or more plastic polymers
into methane and/or liquid fuels is a catalytic process that converts plastic polymers
directly into methane and/or liquid fuels, such as cyclohexane and methylcyclohexane,
and thereby producing value-added gaseous-phase and water immicible products. The
method relies on a heterogeneous catalyst (Ru nanoclusters stabilized by zeolites).
The catalyst is robust and operates with water as a solvent to afford a ready-to-use
hydrogen-enriched methane gas and/or liquid fuels, such as cyclohexane and methylcyclohexane.
The catalyst is also easily recovered and recycled.
[0018] An aspect of the present invention provides a method for preparing a ruthenium-modified
zeolite catalyst for direct conversion of one or more plastic polymers into methane
and/or liquid fuel comprising
- a) providing a zeolite
- b) preparing a mixture of ruthenium (Ru) salt and the zeolite of step a) in a solvent;
- c) stirring the mixture of step b) at 20 to 100°C and cooling the mixture to 20 to
25°C;
- d) adding NaBH4(aq) in the mixture of step c);
- e) stirring the mixture of step d);
- f) recovering ruthenium-modified zeolite catalyst.
[0019] In preferred embodiments, the zeolite is a faujasite type x zeolite (FAU-zeolite)
or zeolite type support having Si/Al ratio from 2-3:1 (type x) to >3:1 (type y) and
pore size from 3-10 Å.
[0020] In preferred embodiments, Ru salt is selected from the group comprising RuCl
3 • xH
2O, [Ru(NH
3)
6]Cl
3, [Ru(NH
3)
5Cl]Cl
2, RuCl
3, RuCl
3 • xH
2O, RuI
3, RuI
3 • H
2O, Ru(NO)(NO
3)
x(OH)
y, x+y=3, RuBr
3, and/or combination thereof.
[0021] In preferred embodiments, solvent is water or alcohol. Preferrably the solvent is
deionized water. Preferred alcohol solvent is methanol or ethanol.
[0022] In some embodiments, the reaction mixture in step c) is stirred at 20 to 80 °C, 20
to 60 °C, 20 to 40 °C; 40 to 100 °C, 60 to 100 °C, 70 to 100 °C, or 70 to 80 °C; preferably
at 70 °C or 75 °C or 80 °C.
[0023] In some embodiments, the reaction mixture in step c) is stirred during suitable period
of time in order to allow incorporation (immobilization, embedding) of ruthenium on
to and/or in to zeolite structure. A suitable period of time is typically 1 to 20
hours, 1 to 18 hours, 1 to 14 hours; 1 to 12 hours, 1 to 8 hours, 1 to 4 hours; 4
to 18 hours, 8 to 18 hours, 12 to 18 hours, or 16 to 18 hours; preferably the suitable
period of time is 1 to 18 hours. Also preferably the suitable period of time is 1
hour, 5 hours, 10 hours, 14 hours, 18 hours or 20 hours.
[0024] In some embodiments, the mixture in step e) is stirred during suitable period of
time in order to allow appropriate reaction (reduction) with NaBH
4. A suitable period of time is typically 0.5 to 5 hours, 0.5 to 3 hours, 0.5 to 2
hours; 0.5 to 1 hour; 1 to 5 hours, 2 to 5 hours; 3 to 5 hours, or 4 to 5 hours; preferably
the suitable period of time is 0.5 to 3 hours. Also preferably the suitable period
of time is 0.5 hour, 1 hour, or 2 hours.
[0025] A cation exchange procedure is used (Figure 1) followed by reduction with excess
NaBH
4(aq) to prepare ruthenium-modified zeolite catalyst, such as Ru-FAU catalyst, of the invention.
The reducing process constructs ruthenium (Ru) nanoparticles (NPs) embedded into the
zeolite framework resulting to the final catalytic solid term ruthenium-modified zeolite,
such as Ru-FAU, Figre 1c). The FAU-zeolite (in the absence of Ru nanoparticles (NPs))
has a particle diameter ranging from 1.5 to 3.0 µm (Figure 2), which remains essentially
the same in the Ru-FAU catalyst (Figure 3a1 vs. a2). HRTEM images reveal that crystalline
Ru NPs protrude from the FAU-zeolite surface with diameters typically ranging from
10 to 20 nm (see figure 3b1 and 3b2 for a comparison between the FAU-zeolite and the
Ru-FAU catalyst). The protruding Ru NPs were further analyzed by high-angle annular
dark-field scanning transmission electron microscopy (HAADF-STEM, Figure 3) together
with STEM-energy-dispersive X-ray spectrometry (STEM-EDX, Figures 4-7). Strong Ru
signals were associated with these structures (Figure 3c1 and c2), whereas Si and
Al are absent within the protruding Ru nanostructure (Figure 3c3 and c4). Elemental
mapping indicates that the pores of the FAU-zeolite are partially filled with Ru (Figure
3c2), firmly embedding the Ru NPs on the support. Brunauer - Emmett - Teller (BET)
analysis showed that the porosity of the FAU-zeolite decreases upon formation of the
Ru NPs. The FAU-zeolite displays a surface area of 423.32 m
2/g (Figure 8) and the Ru-FAU catalyst has a similar adsorption curve, i.e. the adsorption
of a microporous material, but with decreased N
2 adsorption and a surface area of 214.45 m
2/g. This decrease in adsorption indirectly confirms that Ru NPs partially fill the
FAU-zeolite pores. Powder X-ray diffraction (powder-XRD) further demonstrates the
crystalline nature of the Ru-FAU catalyst (Figure 8b) and showed that the native structure
of the FAU support is conserved. Broadening of the peaks combined with a decrease
in peak intensity suggests that the different crystalline frameworks are entangled
and slightly amorphous. X-ray photoelectron spectroscopy (XPS) revealed the presence
of Ru species at 4.4% ± 0.1% on the surface of the Ru-FAU catalyst. (Ru(3p) was selected
to represent the elemental concentration). As expected, aluminum silicate is the major
component of both solids (Table 1).
Table 1. XPS elemental compositions
Cat. |
O(1s) |
Na(1s) |
Mg(2s) |
Al(2p) |
Si(2p) |
Ru(3p) |
FAU-zeolite |
65.3% |
7.9% |
3.5% |
6.5% |
16.7% |
0.1% |
Ru-FAU |
68.2% |
4.6% |
1.1% |
8.3% |
13.4% |
4.4% |
[0026] Note the signal of Na (1s, 1071.50 eV) was chosen as the calibration due to the lack
of suitable C(1s) species. Mg
2+ ions are also present in the support material. The native cations (Na
+ and Mg
2+) are observed by XPS before and after ion exchange indicating that the exchange process
is not quantitative under the conditions used herein (Table 1). The metal loadings
were further examined by means of ICP-OES analysis with 7.3 wt% of Ru, 8.2 wt% of
Na and 0.9 wt% of Mg determined.
[0027] Another aspect of the present invention provides a ruthenium-modified catalyst obtained
by the method of the present invention.
[0028] A further aspect of the present invention provides a use of the ruthenium-modified
zeolite catalyst obtained by the method of the present invention for direct conversion
of one or more plastic polymers into methane and/or liquid fuel. In a preferred embodiment,
the direct conversion of one or more plastic polymers into methane and/or liquid fuel
is according to the method of the present invention.
[0029] Another aspect of the present invention provides a method for converting one or more
plastic polymers into methane and/or liquid fuels comprising
- a) contacting one or more plastic polymers with a ruthenium-modified zeolite catalyst
in water to provide a reaction mixture;
- b) placing the reaction mixture under the pressure of 40 to 200 bars of H2 at 140 to 300 °C, preferably at 200 to 300 °C, most preferably 200 to 250°C;
- c) cooling the reaction mixture to 20 to 25 °C;
- d) recovering methane from the reaction mixture; and/or
- e) recovering liquid fuels from the reaction mixture.
[0030] In preferred embodiments, the water is selected from the group comprising deinoized
water, wastewater, seasalt water, tap water, river water, lake water. Most preferably
the water is deinoized water.
[0031] In preferred embodiments, one or more plastic polymers are selected from the group
comprising polyolefins, ethylene vinyl alcohol (EVOH), poly lactic acid (PLA), polyethylene
terephthalate (PET), polybutylene terephthalate (PBT), polytrimethylene terephthalate
(PTT), polyethylene isosorbide terephthalate (PEIT), polyethylene furanoate (PEF),
polyamide (PA), polyamide-6 or Poly(e-caprolactam) or polycaproamide (PA6), polyamide-6,6
or Poly(hexamethylene adipamide) (PA6,6), Poly(11-aminoundecanoamide) (PA11), polydodecanolactam
(PA 12), poly(tetramethylene adipamide) (PA4,6), poly(pentamethylene sebacamide) (PA5,10),
polyhexamethylene nonanediamideaamide (PA6,9), poly(hexamethylene sebacamide) (PA6,10),
poly(hexamethylene dodecanoamide) (PA6,12), poly(m-xylylene adipamide) (PAMXD6), polyhexamethylene
adipamide/polyhexamethyleneterephtalamide copolymer (PA66/6T), polyhexamethylene adipamide/polyhexamethyleneisophtalamide
copolymer (PA66/6I) polyurethane (PU), polyvinyl chloride (PVC), polyethylene (PE),
polystyrene (PS), acrylonitrile butadiene styrene (ABS), poly(oxide phenylene) (PPO),
polycarbonate (PC), copolymer of phosphono and carboxylic acid (PCA), high molecular
weight polyacrylate, polymethacrylate methyle (PMMA), polyoxymethylene (POM), styrene
acrylonitrile (SAN), polyester polymer alloy (PEPA), polyethylene naphthalate (PEN),
styrene-butadiene (SB) and blends/mixtures of these materials.
[0032] In a particular embodiment, the polyolefin is selected from the group consisting
of polyethylene, polypropylene, polymethylpentene, polybutene-1, polyisobutylene,
ethylene propylene rubber, ethylene propylene diene monomer rubber.
[0033] In a particular embodiment, the polyethylene is divided into low-density and high-density
polyethylene (LDPE and HDPE, respectively).
[0034] In another particular embodiment, the one or more plastic polymers is a polyester
polymer, preferably selected from the group consisting of poly lactic acid (PLA),
polyethylene terephthalate (PET), polybutylene terephthalate (PBT), polytrimethylene
terephthalate (PTT), polyethylene isosorbide terephthalate (PEIT), polyethylene furanoate
(PEF).
[0035] In a particular embodiment, the one or more plastic polymers is a polyamide polymer,
preferably selected from the group consisting of polyamide-6 or poly(e-caprolactam)
or polycaproamide (PA6), polyamide-6,6 or poly(hexamethylene adipamide) (PA6,6), poly(11-aminoundecanoamide)
(PA11).
[0036] In a particular embodiment, the one or more plastic polymers further comprises at
least one polymer selected from the group consisting of aliphatic polyester, polyvinyl
alcohol, cellulose, polylactic acid (PLA), polyhydroxyalkanoate (PHA), starch-based
polymers, poly(butylene adipate-co-terephthalate) (PBAT), polybutylene succinate (PBS),
polybutylene succinate adipate (PBSA), and polycaprolactone (PCL).
[0037] Preferably one or more plastic polymers comprise C=O moiety.
[0038] The one or more plastic polymers can be found in textile fibers, plastic tubes, plastic
films, plastic sheets, or plastic packaging such as flasks, bottles, blister packs,
etc. In some embodiments, the one of more plastic is not pretreated (no pretreatment
is required, the plastic polymer as such is put in contact with the ruthenium-modified
catalyst). In other embodiments, the one or more plastic can undergo a pretreatment,
such as as cutting, crushing, chemical pretreatment, etc....
[0039] The main gaseous product obtained by the method of the invention is methane with
traces of CO
2 (1%) detected. The gas phase also contains unreacted H
2. For example, methane can be recovered from the reaction mixture (step d) by venting
the reactor in the case of a batch reactor setup. Methane is a natural gas widely
used in many industries both as a feedstock for chemical synthesis and as a major
source of electricity generation through the use of gas and steam turbines. Natural
gas burns cleaner than other fossil fuels, such as oil and coal, and produces less
greenhouse gas per unit energy released. Power generation using natural gas is thus
the cleanest fossil fuel source of energy available and this technology is used wherever
competitive.
[0040] Liquid fuels are combustible or energy-generating hydrocarbon molecules, selected
from the group comprising alkanes (such as paraffins, isoparaffins, n-hexane), cycloalkanes
(such as cyclohexane and methylcyclohexane), alkenes, aromatic hydrocarbons (arenes)
and/or combination thereof. In preferred embodiments, the liquid fuels are cyclic
and linear hydrocarbons, most preferably cyclohexane, methylcyclohexane, n-hexane
and/or combination thereof.
[0041] The liquid phase of the reaction mixture contains the initial water and immicible
liquid fuels. Typically, the liquid fuel is recovered from the reaction mixture by
distillation or by recovering the top (oil) layer that is formed.
[0042] As used herein, the ruthenium-modified zeolite is a zeolite that incorporates ruthenium
(Ru) in its structure. The ruthenium-modified zeolite can be typically prepared by
a cation exchange method followed by a chemical reduction by NaBH
4. In some preferred embodiments, the zeolite present in the ruthenium-modified zeolite
catalyst is microporous/mesoporous metallosilicate that include frameworks of type
MWW, MFI, LTL, MOR, BEA, TON, MTW, MTT, FER, MRE, MFS, MEL, DDR, EUO, and FAU (such
as zeolite beta, mordenite, faujasite, Zeolite L, ZSM-5, ZSM-11, ZSM-22, ZSM-23, ZSM-35,
ZSM-48, ZSM-50, ZSM-57, ZSM-58, and MCM-22 family materials) where one or more metals
from groups 8, 11, and 13 of the Periodic Table of the Elements (preferably one or
more of Fe, Cu, Ag, Au, B, Al, Ga, and/or In) are incorporated in the crystal structure
during synthesis or impregnated post crystallization. It is recognized that a metallosilicate
may have one of more metals present and, for example, a material may be referred to
as a ferrosilicate, but it will most likely still contain small amounts of aluminum.
In other preferred embodiments, the zeolite present in the ruthenium-modified zeolite
catalyst is a faujasite type x zeolite (FAU-zeolite) or zeolite type support having
Si/Al ratio from 2-3:1 (type x) to >3:1 (type y) and pore size from 3-10 Å.
[0043] In othere preferred embodiments, the ruthenium-modified zeolite catalyst is doped
with one or more metals selected from the group comprising molybdenum (Mo), rhenium
(Re), zirconium (Zr), zinc (Zn), tungsten (W), Cobalt (Co), Iridium (Ir), Nickel (Ni).
Doping of the catalyst can enhance catalytic activity by means of activation of either
the substrate (here the plastic) and/or the H
2.
[0044] The ruthenium-modified zeolite catalyst can be created with variable meso-/microporosity,
with zeolite porosity optimized for methane and/or liquid fuels conversion, for example,
using a dual-template assisted synthesis method or a hydrothermal method.
[0045] The influence of hydrogen pressure was studied with the conversion of plastic polymer
ranging from 54% at 50 bar to 99% at 100 bar of hydrogen. Applied hydrogen pressure
strongly impacts on the selectivity of gaseous products, with less CO
2 formed at higher hydrogen pressures. This indicates that either hydrolysis is suppressed
or CO
2 is reduced via a Sabatier-type pathway under the reaction conditions. In this respect,
a reaction using CO
2 as substrate demonstrated that Ru-FAU is also able to catalyze the methanation of
CO
2 at temperatures well below those typically used in the Sabatier process. Remarkably,
higher hydrogen pressure also favored the conversion of plastic polymers to gaseous
products. In some embodiments, the hydrogen pressure is 40 to 200 bars, 40 to 150
bars, or 40 to 100 bars, preferably 50 to 100 bars, most preferably 70 to 100 bars.
In other embodiments, the hydrogen pressure is 40 bars, 50 bars, 60 bars, 70 bars,
80 bars, 90 bars, 100 bars; 110 bars, 120 bars, 130 bars, 140 bars, 150 bars, 160
bars, 170 bars, 180 bars, 190 bars, 200 bars; preferably 70 bars or 100 bars.
[0046] In some embodiments, the reaction mixture is placed under the pressure and temperature
according to step b) during a suitable period of time to allow sufficient interaction
of one or more plastic polymers with the ruthenium-modified zeolite catalyst. The
suitable period of time depends on temperature, pressure, ratio between plastic polymers
and catalyst, etc... The suitable period of time is typically 1 to 24 hours, 1 to
20 hours, 1 to 18 hours, 1 to 16 hours, 1 to 14 hours, 1 to 12 hours, 1 to 10 hours,
1 to 8 hours, 1 to 6 hours; 5 to 24 hours, 5 to 20 hours, 5 to 18 hours, 5 to 16 hours,
5 to 14 hours, 5 to 12 hours, 5 to 10 hours, 5 to 8 hours, 5 to 6 hours; 8 to 20 hours,
10 to 20 hours, 12 to 20 hours, 14 to 20 hours, 16 to 20 hours, or 18 to 20 hours.
Preferably, the suitable period of time is 5 to 20 hours or 10 to 20 hours or 10 to
24 hours. Also preferably, the suitable period of time is 10 hours, 18 hours, 18.5
hours, or 20 hours. The step b) can be also a continuous process, wherein at regular
or selected time intervals a portion of the reaction mixture is withdrawn and cooled
according to step c).
[0047] The advantages of the method of the present invention are that the reaction can be
conducted in water. No extra solid residue is observed in the resulting liquid-solid
mixtures (very highly active catalyst, no apparent deactivation). The solid catalyst
is easily recycled since there are no solid by-products or solid resudials.
[0048] This type of conversion is advantageous compared to the current measures which deal
with plastic wastes (burning or limited recycled usages) because the plastics are
converted into fuel chemicals without the release of toxic compounds. Moreover, the
fuel becomes "transportable" (i.e. chemicals are generated and not heat).
[0049] Those skilled in the art will appreciate that the invention described herein is susceptible
to variations and modifications other than those specifically described. It is to
be understood that the invention includes all such variations and modifications without
departing from the spirit or essential characteristics thereof. The invention also
includes all of the steps, features, compositions and compounds referred to or indicated
in this specification, individually or collectively, and any and all combinations
or any two or more of said steps or features. The present disclosure is therefore
to be considered as in all aspects illustrated and not restrictive, the scope of the
invention being indicated by the appended Claims, and all changes which come within
the meaning and range of equivalency are intended to be embraced therein.
[0050] The foregoing description will be more fully understood with reference to the following
Examples. Such Examples, are, however, exemplary of methods of practising the present
invention and are not intended to limit the scope of the invention.
Examples
[0051] All reagents and solvents were purchased from commercial suppliers and used without
further purification. Molecular sieve 10 Å (FAU-zeolite) is a commercially (ROTH)
available Faujasite type X zeolite with general chemical composition Na
86[(AlO
2)
86-(SiO
2)
106] • xH
2O (composition given by the supplier). A Parr stainless-steel autoclave was used for
high-pressure experiments (75 mL capacity). The Brunauer - Emmett - Teller (BET) measurements
were performed on a Belsorp-max gas adsorption analyzer using N
2 as the adsorbate at 77 K. Gas chromatography-mass spectrometry (GC-MS) of liquid
samples was performed on an Agilent 7890B Gas Chromatograph together with Agilent
7000C MS triple quad detector using He as the carrier gas. GC of gaseous samples were
injected manually and detected by Agilent 7890B Gas Chromatograph together with the
kit FID detector using N
2 as the carrier gas. A reference gas bottle for calibration was purchased from Air
Liquide.
1H NMR spectra were recorded on a Bruker 400 MHz instrument. Scanning electron microscopy
(SEM) images were obtained using a Carl Zeiss Gemini 300 microscope. Transmission
electron microscopy (TEM) images and scanning transmission electron microscopy (STEM)
mappings were conducted on a FEI Tecnai Osiris microscope. X-ray photoelectron spectroscopy
XPS measurements were obtained on a PHI VersaProbe II scanning XPS microprobe. X-ray
powder diffraction (XRD) patterns were measured on a Bruker D8 Discover. Inductively
coupled plasma-optical emission spectrometry (ICP-OES) analysis were conducted on
a ICP-OES 5100 from Agilent. Samples were digested in Aqua regia and filtered prior
to measurement.
Example 1 - Preparation of the Ru-FAU catalyst
[0052] Commercial granules of FAU-zeolite (10.0 g) were grounded to powder using a ceramic
mortar. The powder was dispersed in deionized water (DI-water, 75.0 mL) in a round
bottle flask (100 mL). The suspension was sonicated for 10 min and suction filtered.
The residual solid FAU-zeolite was washed with DI-water (3 × 50.0 mL) and dried at
250 °C in an oven for 18 h. RuCl
3 • xH
2O (207 mg, 1.0 mmol) and the FAU-zeolite (1.0 g) were mixed with DI-water (24.0 mL)
in a round bottle flask (100 mL). The suspension was stirred (350 rpm) at 75 °C for
18 h and cooled to room temperature. NaBH
4(s) (189 mg, 5.0 mmol) was dissolved in DI-water (24.0 mL), and added to the round
bottle flask in one portion. The suspension was stirred (800 rpm) for 1 h. The solid
was vacuum filtered and then washed with DI-water (3 × 25 mL). The washed Ru-FAU catalyst
was dried at 250 °C in an oven for 18 h.
Schematic representation of the Ru-FAU catalyst preparation:
[0053] 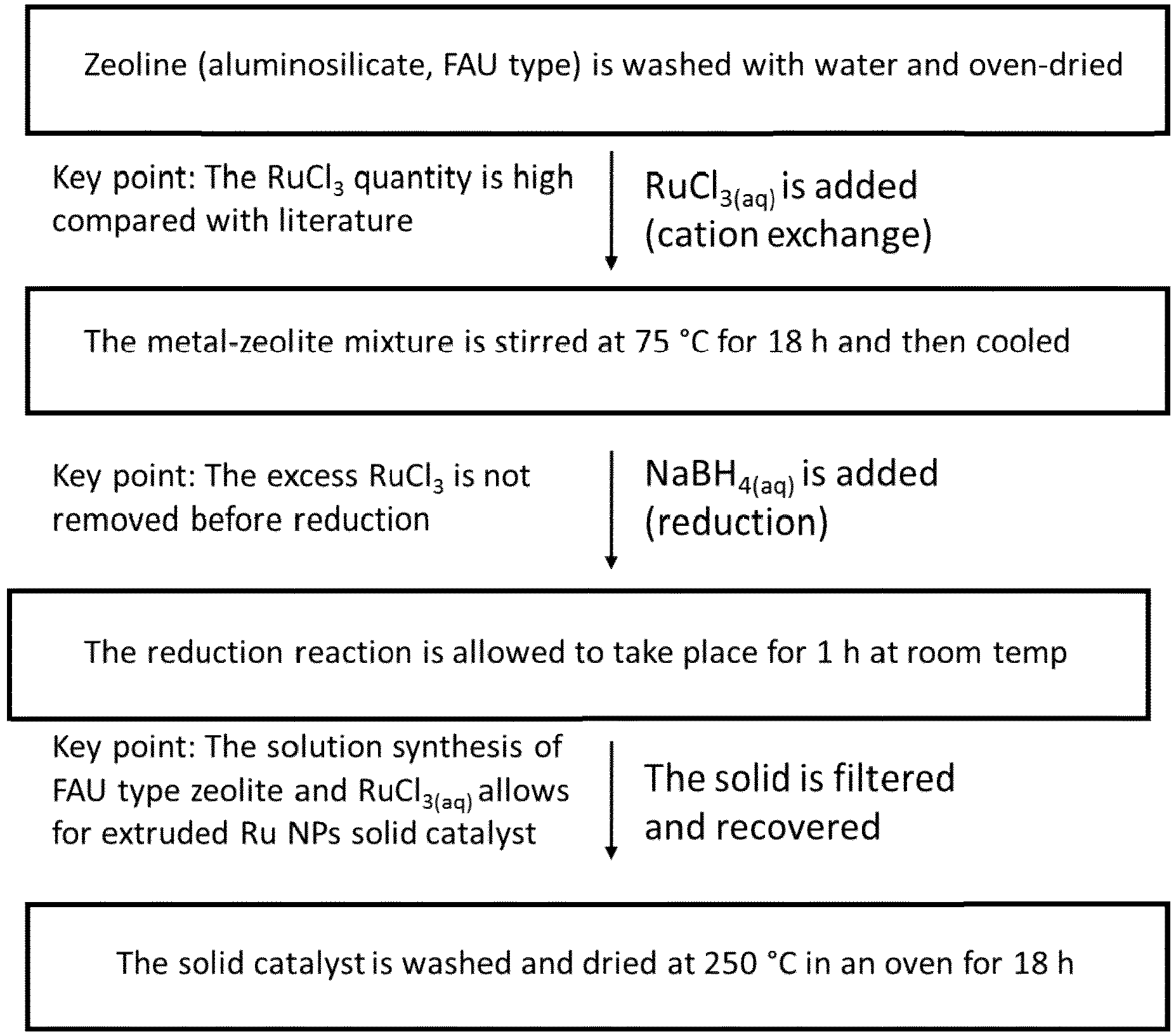
Example 2 - Catalyst robustness
[0054] To evaluate the robustness of the catalyst, recycling experiments were performed
with a total of 5 catalytic cycles of the Ru-FAU catalyst demonstrating that the methane
yields remain almost constant (Table 2). Propylene carbonate (a cyclic carbonate compound)
was used in these recycling studies.
Table 2. Recycling of the Ru-FAU catalyst
Cycle |
Conv. [%] of propylene carbonate |
Gas-products [%] |
CH4 |
CO2 |
C3H8 |
1 |
98 |
24 |
1 |
2 |
2 |
99 |
42 |
1 |
5 |
3 |
99 |
50 |
2 |
7 |
4 |
99 |
49 |
3 |
11 |
5 |
99 |
49 |
3 |
11 |
[0055] As a comparison, a commercial Ru/C was tested, which led to 69% conversion of propylene
carbonate (PC) and produced methane and other products, but upon recycling the activity
decreased markedly and methane was obtained in a lower yield (Table 3). The FAU-zeolite
or RuCl
3 alone are able to convert PC, but are not selective towards glycol and methane. No
conversion was achieved by these three catalysts under nitrogen, confirming that the
potential presence of water in the catalyst does not participate in the hydrolysis
of the cyclic carbonate.
Table 3. Control experiments
Entry |
Catalyst |
Press. (bar) |
Conv. [%] |
Liquid-products [%] |
Gas-products [%] |
PG |
CH4 |
CO2 |
C3H8 |
1 |
RuCl3 |
70 (N2) |
< 1 |
< 1 |
N.R. |
< 1 |
N.R. |
2 |
FAU-zeolite |
70 (N2) |
< 5 |
< 4 |
N.R. |
< 1 |
N.R. |
3 |
Ru/C |
70 (N2) |
< 2 |
< 2 |
N.R. |
1 |
N.R. |
4 |
Ru/C |
70 (H2) |
69 |
14 |
43 |
4 |
9 |
5 |
Ru/C |
70 (H2) |
41 |
30 |
1 |
9 |
1 |
6 |
Cu-FAU |
70 (H2) |
20 |
10 |
N.R. |
5 |
N.R. |
7 |
FAU-zeolite |
70 (H2) |
34 |
7 |
1 |
6 |
N.R |
8 |
RuCl3 |
70 (H2) |
54 |
19 |
N.R |
6 |
N.R |
Example 3 - Plastic polymer conversion
[0056] Plastic (2.5 mmole, M.W. = repeating unit) and Ru-FAU (50 mg) were added to a microwave
reaction vial (10 mL) and capped. The cap was pierced with a needle and the vial was
inserted into an autoclave (75 mL). The autoclave was purged 3 times with H
2 and then pressurized to 100 bars of H
2. The pressurized autoclave was placed in a heating block at 200 ºC and stirred (750
rpm) for 18.5 h. After the reaction, the autoclave was cooled to room temperature
in a water bath. The gaseous products were transferred into a balloon and injected
into a GC for analysis. The liquid phase was extracted with dichloromethane (3 mL).
The water layer analyzed by
1H NMR spectroscopy. The organic layer was separated and dried by a rotary evaporator.
The dry products were re-dissolved in d6-acetone and analyzed by NMR spectroscopy.
Results:
[0057]
Structure |
Mg. |
Solid Residual [%] |
Conv. [%] |
CH4 [%] |
Cyclohexane [%] |
Methyl-cyclehexane [%] |
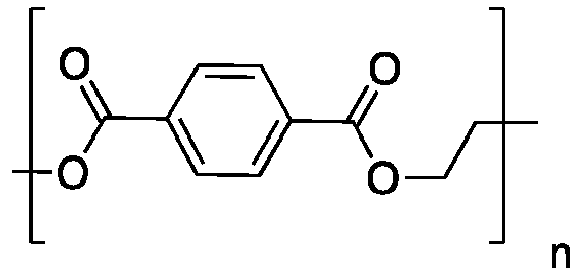
|
480 |
∼1 |
∼100 |
52 |
16 |
8 |
1. A method for converting one or more plastic polymers into methane and/or liquid fuels
comprising
a) contacting one or more plastic polymers with a ruthenium-modified zeolite catalyst
in water to provide a reaction mixture;
b) placing the reaction mixture under the pressure of 40 to 200 bars of H2 at 140 to 300°C;
c) cooling the reaction mixture to 20 to 25°C;
d) recovering methane from the reaction mixture; and/or
e) recovering liquid fuels from the reaction mixture.
2. The method of claim 1, wherein the temperature in step b) is 200 to 300 °C or 200
to 250 °C.
3. The method of claim 1 or 2, wherein the water is selected from the group comprising
deinoized water, wastewater, seasalt water, tap water, river water, lake water.
4. The method of any one of claims 1 to 3, wherein one or more plastic polymers are selected
from the group comprising polyolefins, ethylene vinyl alcohol (EVOH), poly lactic
acid (PLA), polyethylene terephthalate (PET), polybutylene terephthalate (PBT), polytrimethylene
terephthalate (PTT), polyethylene isosorbide terephthalate (PEIT), polyethylene furanoate
(PEF), polyamide (PA), polyamide-6 or Poly(e-caprolactam) or polycaproamide (PA6),
polyamide-6,6 or Poly(hexamethylene adipamide) (PA6,6), Poly(11-aminoundecanoamide)
(PA11), polydodecanolactam (PA 12), poly(tetramethylene adipamide) (PA4,6), poly(pentamethylene
sebacamide) (PA5,10), polyhexamethylene nonanediamideaamide (PA6,9), poly(hexamethylene
sebacamide) (PA6,10), poly(hexamethylene dodecanoamide) (PA6,12), poly(m-xylylene
adipamide) (PAMXD6), polyhexamethylene adipamide/polyhexamethyleneterephtalamide copolymer
(PA66/6T), polyhexamethylene adipamide/polyhexamethyleneisophtalamide copolymer (PA66/6I)
polyurethane (PU), polyvinyl chloride (PVC), polyethylene (PE), polystyrene (PS),
acrylonitrile butadiene styrene (ABS), poly(oxide phenylene) (PPO), polycarbonate
(PC), copolymer of phosphono and carboxylic acid (PCA), high molecular weight polyacrylate,
polymethacrylate methyle (PMMA), polyoxymethylene (POM), styrene acrylonitrile (SAN),
polyester polymer alloy (PEPA), polyethylene naphthalate (PEN), styrene-butadiene
(SB) and blends/mixtures of these materials.
5. The method of any one of claims 1 to 4, wherein the liquid fuels are combustible or
energy-generating hydrocarbon molecules, selected from the group comprising alkanes,
cycloalkanes, alkenes, aromatic hydrocarbons and/or combination thereof.
6. The method of any one of claims 1 to 5, wherein the liquid fuels selected from the
group comprising cyclohexane, methylcyclohexane, n-hexane and/or combination thereof.
7. The method of any one of claims 1 to 6, wherein the ruthenium-modified zeolite catalyst
is doped with one or more metals selected from the group comprising molybdenum (Mo),
rhenium (Re), zirconium (Zr), zinc (Zn), tungsten (W), Cobalt (Co), Iridium (Ir),
Nickel (Ni).
8. A method for preparing a ruthenium-modified zeolite catalyst for direct conversion
of one or more plastic polymers into methane and/or liquid fuel comprising
a) providing a zeolite
b) preparing a mixture of ruthenium (Ru) salt and the zeolite of step a) in a solvent;
c) stirring the mixture of step b) at 20 to 100 °C and cooling the mixture to 20 to
25°C;
d) adding NaBH4(aq) in the mixture of step c);
e) stirring the mixture of step d);
f) recovering ruthenium-modified zeolite catalyst.
9. The method of claim 8, wherein the zeolite is a faujasite type x zeolite (FAU-zeolite)
or zeolite type support having Si/Al ratio from 2-3:1 (type x) to >3:1 (type y) and
pore size from 3-10 Å.
10. The method of claims 8 or 9, wherein Ru salt is selected from the group comprising
RuCl3 • x H2O, [Ru(NH3)6]Cl, [Ru(NH3)5Cl]Cl2, RuCl3, RuCl3 • xH2O, RuI3, RuI3 • H2O, Ru(NO)(NO3)x(OH)y, x+y=3, RuBr3, and/or combination thereof.
11. The method of any one of claims 8 to 10, wherein the solvent is water or alcohol,
preferrably deionized water.
12. A ruthenium-modified catalyst obtained by the method of any one of claims 8 to 11.
13. Use of the ruthenium-modified zeolite catalyst obtained by the method of any one of
claims 8 to 11 for direct conversion of one or more plastic polymers into methane
and/or liquid fuel.
14. The use of claim 13, wherein the direct conversion of one or more plastic polymers
into methane and/or liquid fuel is according to the method of claims 1 to 7.